Kennedy Space Center – After overcoming a frightening thruster failure that could have spelled rapid doom on the heels of a breathtakingly beautiful launch, the privately developed Dragon spacecraft successfully berthed at the International Space Station (ISS) a short while ago, at 8:56 a.m. EST Sunday morning, March 3, 2013 – thereby establishing an indispensable American Lifeline to the massive orbiting lab complex.
Hearts sank and hopes rose in the span of a few troubling hours following Friday’s (Mar. 1) flawless launch of the Dragon cargo resupply capsule atop the 15 story tall Falcon 9 rocket from Cape Canaveral Air Force Station, Florida and the initial failure of the life giving solar arrays to deploy and failure of the maneuvering thrusters to fire.
“Congrats to the @NASA/@SpaceX team. Great work getting #Dragon to the #ISS…our foothold for future exploration!” tweeted NASA Deputy Administrator Lori Garver.
Space station Expedition 34 crew members Kevin Ford and Tom Marshburn of NASA used the station’s 58 foot long Canadian supplied robotic arm to successfully grapple and capture Dragon at 5:31 a.m. Sunday as the station was flying 253 miles above northern Ukraine. See the grappling video – here.
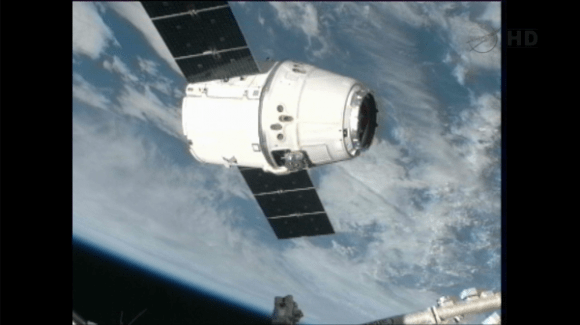
“The vehicle’s beautiful, space is beautiful, and the Canadarm2 is beautiful too”, said station commander Kevin Ford during the operation.
The capsule pluck from free space came one day, 19 hours and 22 minutes after the mission’s launch.
Ground controllers at NASA’s Johnson Space Center in Houston then commanded the arm to install Dragon onto the Earth-facing port of the Harmony module – see schematic.

Originally, Dragon capture was slated only about 20 hours after launch. But that all went out the window following the serious post-launch anomalies that sent SpaceX engineers desperately scrambling to save the flight from a catastrophic finale.
The $133 million mission dubbed CRS-2 is only the 2nd contracted commercial resupply mission ever to berth at the ISS under NASA’s Commercial Resupply Services (CRS) contract. The contract is worth $1.6 Billion for at least a dozen resupply flights.
Following the forced retirement of NASA’s space shuttle orbiters in July 2011, American was left with zero capability to launch either cargo or astronauts to the primarily American ISS. NASA astronauts are 100% reliant on Russian Soyuz capsules for launch to the ISS.
Both the Falcon 9 rocket and Dragon spacecraft were designed and built by SpaceX Corporation based in Hawthorne, Calif., and are entirely American built.
The Falcon 9/Dragon commercial system restores America’s unmanned cargo resupply capability. But the time gap will be at least 3 to 5 years before American’s can again launch to the ISS aboard American rockets from American soil.
And continuing, relentless cuts to NASA’s budget are significantly increasing that human spaceflight gap and consequently forces more payments to Russia.
“Today we marked another milestone in our aggressive efforts to make sure American companies are launching resupply missions from U.S. shores,” said NASA Admisistrator Charles Bolden in a NASA statement.
“Our NASA-SpaceX team completed another successful berthing of the SpaceX Dragon cargo module to the International Space Station (ISS) following its near flawless launch on the Falcon-9 booster out of Cape Canaveral, Florida Friday morning. Launching rockets is difficult, and while the team faced some technical challenges after Dragon separation from the launch vehicle, they called upon their thorough knowledge of their systems to successfully troubleshoot and fully recover all vehicle capabilities. Dragon is now once again safely berthed to the station.”
“I was pleased to watch the launch from SpaceX’s facility in Hawthorne, CA, and I want to congratulate the SpaceX and NASA teams, who are working side by side to ensure America continues to lead the world in space.”
“Unfortunately, all of this progress could be jeopardized with the sequestration ordered by law to be signed by the President Friday evening. The sequester could further delay the restarting of human space launches from U.S. soil, push back our next generation space vehicles, hold up development of new space technologies, and jeopardize our space-based, Earth observing capabilities,” said Bolden.
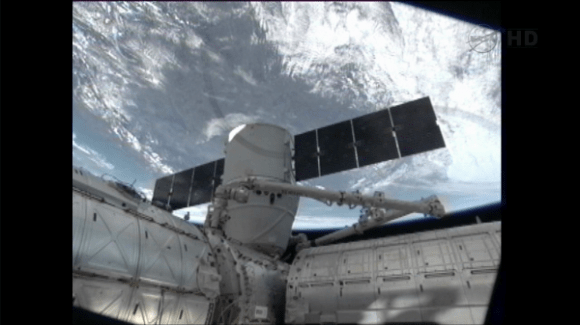
Dragon is loaded with about 1,268 pounds (575 kilograms) of vital supplies and provisions to support the ongoing science research by the resident six man crew, including more than a ton of vital supplies, science gear, research experiments, spare parts, food, water and clothing.
NASA says that despite the one-day docking delay, the Dragon unberthing will still be the same day as originally planned on March 25 – followed by a parachute assisted splashdown in the Pacific Ocean off the coast of Baja California.
Dragon will spend 22 days docked to the ISS. The station crew will soon open the hatch and unload all the up mass cargo and research supplies. Then they will pack the Dragon with about 2,668 pounds (1,210 kilograms) of science samples from human research, biology and biotechnology studies, physical science investigations, and education activities for return to Earth.
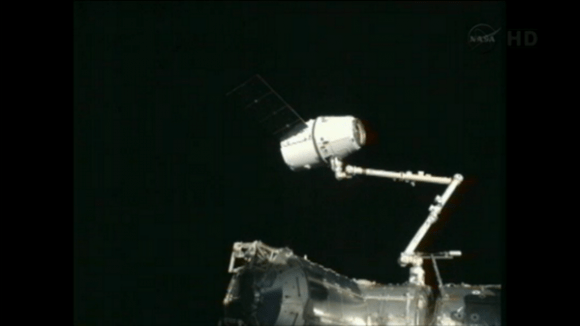
Dragon is the only spacecraft in the world today capable of returning significant amounts of cargo to Earth.
Orbital Sciences Corp also won a $1.9 Billion cargo resupply contract from NASA to deliver cargo to the ISS using the firm’s new Antares rocket and Cygnus capsule.
NASA hopes the first Antares/Cygnus demonstration test flight from NASA’s Wallops Island Facility in Virginia will follow in April. Cygnus cargo transport is one way – to orbit only.
“SpaceX is proud to execute this important work for NASA, and we’re thrilled to bring this capability back to the United States,” said Gwynne Shotwell, President of SpaceX.
“Today’s launch continues SpaceX’s long-term partnership with NASA to provide reliable, safe transport of cargo to and from the station, enabling beneficial research and advancements in technology and research.”
The SpaceX CRS-3 flight is slated to blast off in September 2013.
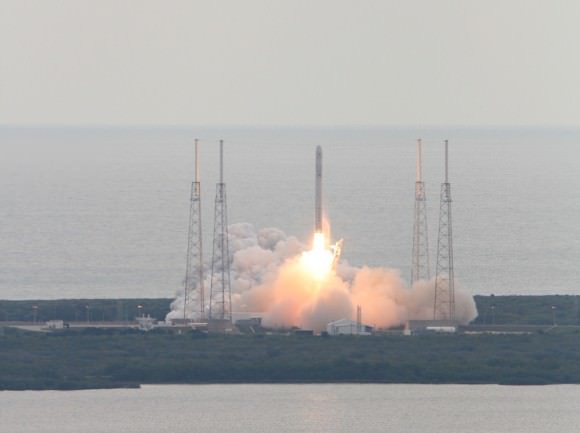
For those interested how a RCS works:
This is from the Apollo lunar lander but the principle of the Dragon should stay the same.
http://ntrs.nasa.gov/archive/nasa/casi.ntrs.nasa.gov/20090016298_2009014419.pdf
Helium in a helium tank is heated up and builds up pressure.
The Helium leads into the oxidizer tank and also in the fuel tank.
There is a membrane in the oxidizer and fuel tank to keep the helium separated form the oxidizer/fuel. Once the fuel and oxidizer is under pressure all you need to do is to open and shut a valve in a precisely timed matter so the oxidizer and fuel can touch each other and spontaneous burn. (In Apollo the fuel is injected in such a way that it creates these cylindrical tubes, the oxidizer was sprayed under a angle to disrupt the cylindrical fuel stream and mix ideally. And since the oxidizer also sprayed against the combustion wall it cooled that part of the engine a bit. Notice the venting valves that would release the too high a pressure into space.
In the case of Apollo, the RCS system was using ablative materials so they had limited burns (minimal 12 ms up to 500 sec). I assume that the Dragon does the same thing.
I have understood that the dragon has each separate helium, oxidizer and fuel tank for each thruster.
As it turns out all of the oxidizer tanks are fed by the same helium pressurization tank, at least according to this source:
http://www.spacesafetymagazine.com/2013/03/02/dragon-glitch-delays-iss-delivery/
This would explain why 3 out of 4 thrusters failed as the root of the problem traces back to a commonly shared element. As Space X decalres on their website that their redundant systems are a safety advantage, it ironically appears that they are not redundant enough.
As the main problem appears to be frozen helium, it would seem that a fix would be as simple as providing better insulation for the helium tank and lines.
…and without spending the “big money” some posters here feel is the only way to correct a problem. 😉
Well said it’s amazing what happens when you wait for some FACTS before jumping to wild unbased conclusions.
On the contrary, this freezing must be added to the test cycle.
Is there room for that insulation? What will the effect of that insulation be at the rest of the installation? If there is a leakage of fuel or oxidizer, will the insulation spontaneous combust? Does this isolation actually work when the rocket is delayed and have to stand another 24h on the launch pad?
Sounds much more complicated than building an LEO launch rocket. You’ve finally figured how to beat SpaceX! 😉
[We don’t know the root cause yet. Speculation is futile.]
Great link. Was not aware of that site, thanks a bunch!
Bit it is still the same problem. 3 worked, 1 failed. If the main helium valve was not working then all 4 thrusters should not have operated.
Unless there was not enough pressure and the helium escaped through the only thruster with the least resistance.
We would all sound more intelligent of we just had their blueprints or at least a simple schematic of their thrusters. If the source I quoted is correct, then it is a little puzzling how the helium froze considering the temperature had to get to 4K for that to happen (-270 celcius). As opposed to 55K (-218 celcius) for oxygen, and as this part of the thruster was operational it did not freeze. Since the helium froze fairly quickly, it makes you wonder what failed for the temperature to drop so low inside the helium tank or it’s connections. To answer this you need to know how their thruster system maintains heat, but there is no way to know this without their blueprints.
As far as I understood, is is not the Helium that froze. It is the oxidizer.
But the oxidizer is not oxygene but NitrogenTetroxide (Hydrazine/NitrogenTetroxide) where Hydrazine is the fuel.
NitrogenTetroxide freezes at ?11.2 °C.
Normally the Helium tank should be thermo dynamical shielded from the oxidizer lines. The helium then gets heated up to avoid freezing the pipes and oxidizer.
This is what the apollo did:
http://ntrs.nasa.gov/archive/nasa/casi.ntrs.nasa.gov/19730060786_1973060786.pdf
“The descent propellant tanks are pressurized by helium that is supercrifically stored as a high density gas in a cryogenic storage vessel and piped through a series of valves and pressure-reducing reylators. The helium is then introduced directly into the propellant tanks, where it acts on the surface 0 of the fluids to force them through the system to the engine. ”
“When the squib is fired, the helium initially passes through the first loop of a two-pass fuel/helium heat exchanger, where it is permitted to absorb heat from the engine fuel, which has ken circulated from the fuel tanks directly to the heat exchanger, before its ultimate delivery to the engine (see figure 3-14). The warmed helium is then routed back through the internal heat exchanger inside the storage vessel; the resultant heat transfer to the re-maw gas in the vessel maintain the continuing pressure required to expel the helium throughout the entire period of operation After passing through the internal heat exchanger, the helium is routed back through the second loop of the fuel/helium heat exchanger and post-heated for temperature conditioning prici.
to delivery to the regulator set”