The first results from the largest and most complex scientific instrument on board the International Space Station has provided tantalizing hints of nature’s best-kept particle secrets, but a definitive signal for dark matter remains elusive. While the AMS has spotted millions of particles of antimatter – with an anomalous spike in positrons — the researchers can’t yet rule out other explanations, such as nearby pulsars.
“These observations show the existence of new physical phenomena,” said AMS principal investigator Samuel Ting,” and whether from a particle physics or astrophysical origin requires more data. Over the coming months, AMS will be able to tell us conclusively whether these positrons are a signal for dark matter, or whether they have some other origin.”
The AMS was brought to the ISS in 2011 during the final flight of space shuttle Endeavour, the penultimate shuttle flight. The $2 billion experiment examines ten thousand cosmic-ray hits every minute, searching for clues into the fundamental nature of matter.
During the first 18 months of operation, the AMS collected of 25 billion events. It found an anomalous excess of positrons in the cosmic ray flux — 6.8 million are electrons or their antimatter counterpart, positrons.
The AMS found the ratio of positrons to electrons goes up at energies between 10 and 350 gigaelectronvolts, but Ting and his team said the rise is not sharp enough to conclusively attribute it to dark matter collisions. But they also found that the signal looks the same across all space, which would be expected if the signal was due to dark matter – the mysterious stuff that is thought to hold galaxies together and give the Universe its structure.
Additionally, the energies of these positrons suggest they might have been created when particles of dark matter collided and destroyed each other.
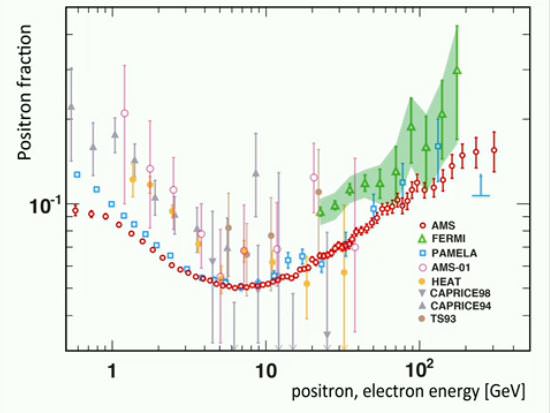
The AMS results are consistent with the findings of previous telescopes, like the Fermi and PAMELA gamma-ray instruments, which also saw a similar rise, but Ting said the AMS results are more precise.
The results released today do not include the last 3 months of data, which have not yet been processed.
“As the most precise measurement of the cosmic ray positron flux to date, these results show clearly the power and capabilities of the AMS detector,” Ting said.
Cosmic rays are charged high-energy particles that permeate space. An excess of antimatter within the cosmic ray flux was first observed around two decades ago. The origin of the excess, however, remains unexplained. One possibility, predicted by a theory known as supersymmetry, is that positrons could be produced when two particles of dark matter collide and annihilate. Ting said that over the coming years, AMS will further refine the measurement’s precision, and clarify the behavior of the positron fraction at energies above 250 GeV.
Although having the AMS in space and away from Earth’s atmosphere – allowing the instruments to receive a constant barrage of high-energy particles — during the press briefing, Ting explained the difficulties of operating the AMS in space. “You can’t send a student to go out and fix it,” he quipped, but also added that the ISS’s solar arrays and the departure and arrival of the various spacecraft can have an effect on thermal fluctuations the sensitive equipment might detect. “You need to monitor and correct the data constantly or you are not getting accurate results,” he said.
Despite recording over 30 billion cosmic rays since AMS-2 was installed on the International Space Station in 2011, the Ting said the findings released today are based on only 10% of the readings the instrument will deliver over its lifetime.
Asked how much time he needs to explore the anomalous readings, Ting just said, “Slowly.” However, Ting will reportedly provide an update in July at the International Cosmic Ray Conference.
More info: CERN press release, the team’s paper: First Result from the Alpha Magnetic Spectrometer on the International Space Station: Precision Measurement of the Positron Fraction in Primary Cosmic Rays of 0.5–350 GeV
Thanks for the update Nancy! Finally… some long awaited results from this experiment! I for one, can hardly wait to hear more! That thing was expensive but is finally beginning to pay off! Any further developments will be greatly appreciated…
For the time being, I’ll gravitate more toward MOND over Dark Matter…
That is rich, considering that first WMAP 9 year and now Planck 4 year data releases independently and conclusively excluded MOND within a few weeks at currently 7-25 (!) sigma, depending on which constraint testing GR within standard cosmology you choose, see the Planck papers.
General relativity won, Flat Earth (“Flat Space”) lost.
[Theorist] Look, I took the liberty of examining that theory when I got it home, and I discovered the only reason that it had been sitting on arxiv in the first place was that it had been NAILED there.
(pause)
[Shop Kook] Well, o’course it was nailed there! If I hadn’t nailed that theory down, it would have nuzzled up to those galactic haloes, bent ’em apart with its ad hoc power law, and VOOM! Feeweeweewee!
[Theorist] “VOOM”?!? Mate, this theory wouldn’t “voom” if you put four million words through it! ‘E’s bleedin’ demised!
[SH]: No no! ‘E’s pining!
[Theorist]: ‘E’s not pinin’! ‘E’s passed on! This theory is no more! He has ceased to be! ‘E’s expired and gone to meet ‘is maker! ‘E’s a stiff! Bereft of life, ‘e rests in peace!
If you hadn’t nailed ‘im to the arxiv ‘e’d be pushing up the daisies! ‘Is scientific processes are now ‘istory! ‘E’s off the twig! ‘E’s kicked the bucket, ‘e’s shuffled off ‘is mortal coil, run down the curtain and joined the bleedin’ choir invisibile!!
THIS IS AN EX-THEORY!!
[With excuses to Monty Python’s Flying University.]
You don’t like my joke, eh? LOL!
Definitely looking forward to more future details on this.
Really nice all around!
– Good news for me, as this is IMHO much easier to get one’s head around than the Fermi-LAT haphazard “peaks”. At 95 % confidence, an isotropic flux from diffusion in the DM halo, see http://physics.aps.org/articles/v6/40 fig. 2 for a nice illustration. And the Fermi-LAT tentative peak is definitely gone within even early error bounds (paper, fig. 5).
– Good news for LHC, if the fraction tapers off towards 1 TeV WIMP (500 GeV positrons). They should be able to detect that.
– Good news for physicists, as a putative ~ 1 TeV supersymmetric WIMP would solve the hierarchy problem. I.e. why the hierarchy of standard particles being so light compared to the Planck energy associated with quantum particles.
– Good news for Ting, having AMS turning out these data.
Planck nailed 5+1 parameter standard cosmology inflation. And its data predicts it is nearly definitively a slow-roll inflation. (I.e. the inflationary process rolling down a small hill, a potential hill which can come from pretty much anything I take it.) Without any fancy extra tensorial gravity waves, except possibly expected scalar ones coming from a local end of inflation making the early universe ring a little from DM and other particles being created at slightly different times.
On such a simple background I would give the best odds for that LHC will find Split Supersymmetry instead of finetuned supersymmetry which needs yet more physics to be predicted out of. I.e. what is unnatural for a “theory of everything” physicist is the most natural for a slow-roll inflation, a dynamics of local freezing out of first supersymmetric particles, then standard particles.
Which likely means an assuredly here and there inhabited multiverse. Wouldn’t it be nice to see the universe in the vacuum tubes of the LHC detector? I think it would be poetic.
Inflation has a curious relationship to supersymmetry. Inflation occurs when the vacuum energy is very large. The stress-energy of the solution is
T^{ab} = (? + p)U^aU^b +g^{ab}p
The energy density ? describes the vacuum energy, which in the inflationary period is very large. For inflation there is the condition on p = w? is w = -1. This means the pressure p is large and negative, which accounts for the enormous expansion rate of the early universe. This means with the four velocities U^aU_a = -1 that T_{ab}U^aU^b = ?, which is greater than zero. The T^{00} = H = ?, or the energy, which is positive and large. Supersymmetry on the other hand is rather fussy about this. The Hamiltonian in supersymmetry is zero H = 0, and the condition H > 0 is the case for broken supersymmetry.
The earlier universe, or quantum cosmology, is then presumably one where H = 0, which would permit supersymmetry, and probably one of these high level supersymmetries N = 4 or 8. Yet this get broken with inflation. Inflation ends with bubble nucleation, which generates a region with a much smaller vacuum energy density. This bubble is one of these Linde-Velinkin pocket universes, and a large number of these on a spacetime manifold of inflation describes one level of the multiverse. Maybe upon this nucleation, which is also called reheating, a lower form of supersymmetry, N = 1 or 2, is recovered, or recovered with a small symmetry breaking, that then breaks at the lower electroweak energy scale.
The prime supersymmetric candidate for dark matter is the neutralino. This is a condensate state of the superpartners of the photon, Z, and higgs particle that all have the same quantum numbers. The Fermi spacecraft has found a peak of energy production at about the energy scale expected for the neutralino. The energy spike in the AMS data is further suggestive of such physics.
LC
Any idea when will we see an update allowing us to know if:
1) the excess persists at higher energies (like in the case that this excess is due to pulsars)
2) will at some point have a drop (as is predicted to happen after one measure positrons over energies bigger than the WIMP dark matter mass)
Months? Years?
That is a beginning of confirmation for the string theory and BB too:
http://gravityattraction.wordpress.com/2013/04/01/boltzmann-brain-discovery/