When is a Brown Dwarf star not a star at all, but only a mere Gas Giant? And when is a Gas Giant not a planet, but a celestial object more akin to a Brown Dwarf? These questions have bugged astronomers for years, and they go to the heart of a new definition for the large celestial bodies that populate solar systems.
An astronomer at Johns Hopkins University thinks he has a better way of classifying these objects, and it’s not based only on mass, but on the company the objects keep, and how the objects formed. In a paper published in the Astrophysical Journal, Kevin Schlaufman made his case for a new system of classification that could helps us all get past some of the arguments about which object is a gas giant planet or a brown dwarf. Mass is the easy-to-understand part of this new definition, but it’s not the only factor. How the object formed is also key.
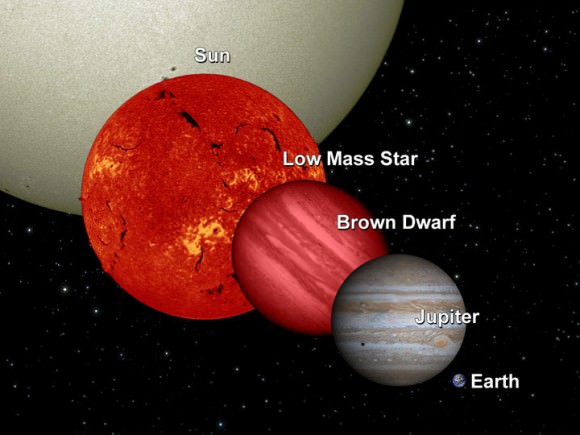
Schlaufman is an assistant professor in the Johns Hopkins Department of Physics and Astronomy. He has set a limit for what we should call a planet, and that limit is between 4 and 10 times the mass of our Solar System’s biggest planet, Jupiter. Above that, you’ve got yourself a Brown Dwarf star. (Brown Dwarfs are also called sub-stellar objects, or failed stars, because they never grew massive enough to become stars.)
“An upper boundary on the masses of planets is one of the most prominent details that was missing.” – Kevin Schlaufman, Johns Hopkins University, Dept. of Physics and Astronomy.
Improvements in observing other solar systems have led to this new definition. Where previously we only had our own Solar System as reference, we now can observe other solar systems with increasing effectiveness. Schlaufman observed 146 solar systems, and that allowed him to fill in some of the blanks in our understanding of brown dwarf and planet formation.
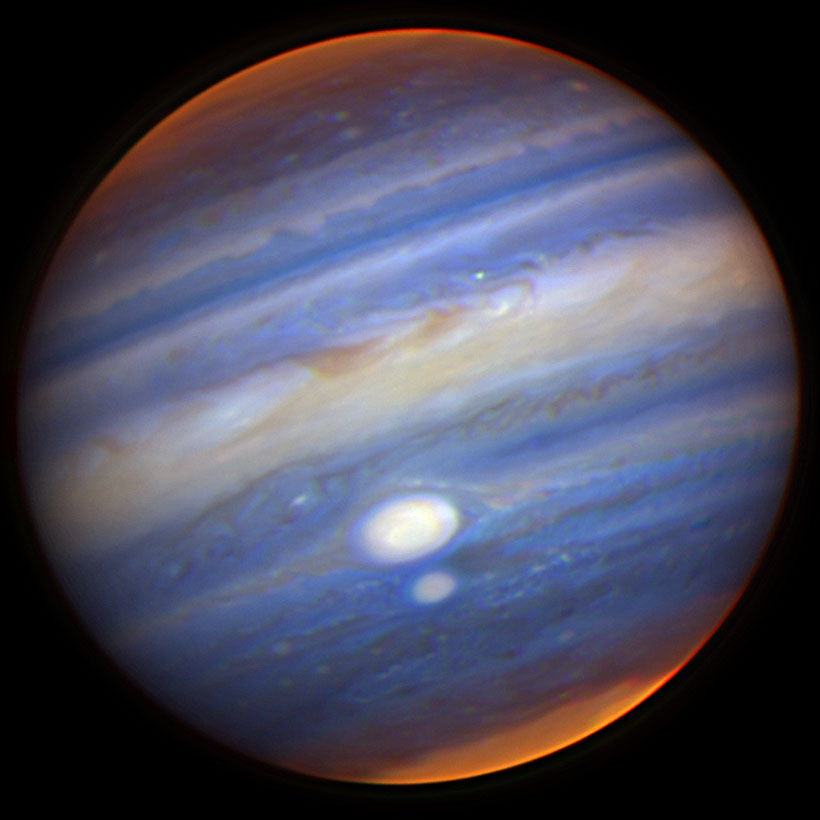
“While we think we know how planets form in a big picture sense, there’s still a lot of detail we need to fill in,” Schlaufman said. “An upper boundary on the masses of planets is one of the most prominent details that was missing.”
Let’s back up a bit and look at how Brown Dwarfs and Gas Giants are related.
Solar systems are formed from clouds of gas and dust. In the early days of a solar system, one or more stars are formed out of this cloud by gravitational collapse. They ignite with fusion and become the stars we see everywhere in the Universe. The leftover gas and dust forms into planets, or brown dwarfs. This is a simplified version of solar system formation, but it serves our purposes.
In our own Solar System, only a single star formed: the Sun. The gas giants Jupiter and Saturn gobbled up most of the rest of the material. Jupiter gobbled up the lion’s share, making it the largest planet. But what if conditions had been different and Jupiter had kept growing? According to Schlaufman, if it had kept growing to over 10 times the size it is now, it would have become a brown dwarf. But that’s not where the new definition ends.
Metallicity and Chemical Makeup
Mass is only part of it. What’s really behind his new classification is the way in which the object formed. This involves the concept of metallicity in stars.
Stars have a metallicity content. In astrophysics, this means the fraction of a star’s mass that is not hydrogen or helium. So any element from lithium on down is considered a metal. These metals are what rocky planets form from. The early Universe had only hydrogen and helium, and almost insignificant amounts of the next two elements, lithium and beryllium. So the first stars had no metallicity, or almost none.
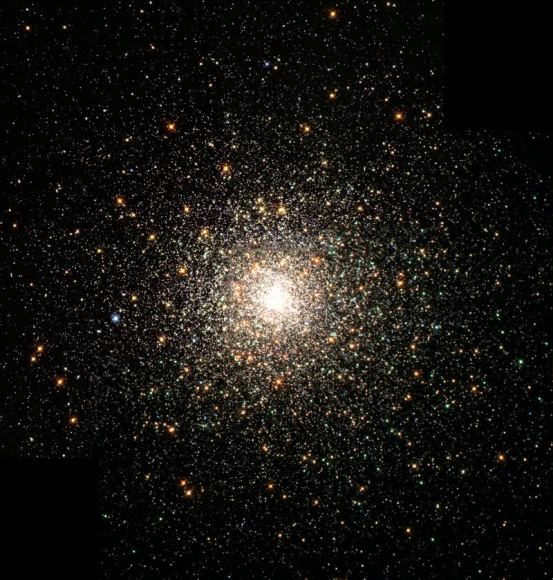
But now, 13.5 billion years after the Big Bang, younger stars like our Sun have more metal in them. That’s because generations of stars have lived and died, and created the metals taken up in subsequent star formation. Our own Sun was formed about 5 billion years ago, and it has the metallicity we expect from a star with its birthdate. It’s still overwhelmingly made of hydrogen and helium, but about 2% of its mass is made of other elements, mostly oxygen, carbon, neon, and iron.
This is where Schlaufman’s study comes in. According to him, we can distinguish between gas giants like Jupiter, and brown dwarfs, by the nature of the star they orbit. The types of planets that form around stars mirror the metallicity of the star itself. Gas giants like Jupiter are usually found orbiting stars with metallicity equal to or greater than our Sun. But brown dwarfs aren’t picky; they form around almost any star. Why?
Brown Dwarfs and Planets Form Differently
Planets like Jupiter are formed by accretion. A rocky core forms, then gas collects around it. Once the process is done, you have a gas giant. For this to happen, you need metals. If metals are present for these rocky cores to form, their presence will be reflected in the metallicity of the host star.
But brown dwarfs aren’t formed by accretion like planets are. They’re formed the same way stars are; by gravitational collapse. They don’t form from an initial rocky core, so metallicity isn’t a factor.
This brings us back to Kevin Schlaufman’s study. He wanted to find out the mass at which point an object doesn’t care about the metallicity of the star they orbit. He concluded that objects above 10 times the mass of Jupiter don’t care if the star has rocky elements, because they don’t form from rocky cores. Hence, they’re not planets akin to Jupiter; they’re brown dwarfs that formed by gravitational collapse.
What Does It Matter What We Call Them?
Let’s look at the Pluto controversy to understand why names are important.
The struggle to accurately classify all the objects we see out there in space is ongoing. Who can forget the plight of poor Pluto? In 2006, the International Astronomical Union (IAU) demoted Pluto, and stripped it of its long-standing status as a planet. Why?
Because the new definition of what a planet is relied on these three criteria:
- a planet is in orbit around a star.
- a planet must have sufficient mass to assume a hydrostatic equilibrium (a nearly round shape.)
- a planet has cleared the neighbourhood around its orbit
The more we looked at Pluto with better telescopes, the more we realized that it did not meet the third criteria, so it was demoted to Dwarf Planet. Sorry Pluto.
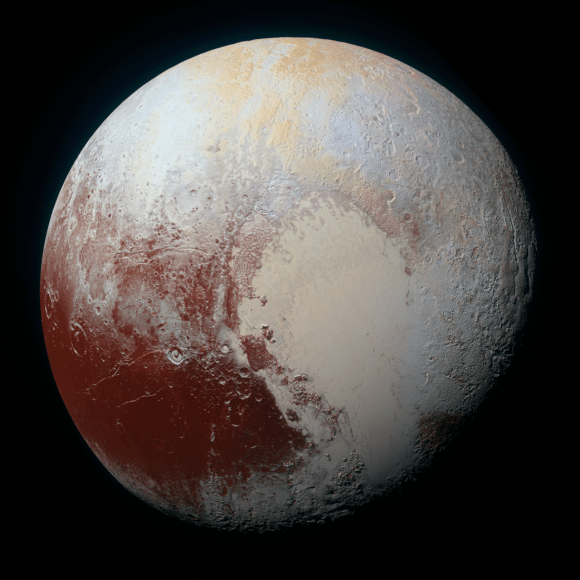
Our naming conventions for astronomical objects are important, because they help people understand how everything fits together. But sometimes the debate over names can get tiresome. (The Pluto debate is starting to wear out its welcome, which is why some suggest we just call them all “worlds.”)
Though the Pluto debate is getting tiresome, it’s still important. We need some way of understanding what makes objects different, and names that reflect that difference. And the names have to reflect something fundamental about the objects in question. Should Pluto really be considered the same type of object as Jupiter? Are both really planets in the same sense? The IAU says no.
The same principle holds true with brown dwarfs and gas giants. Giving them names based solely on their mass doesn’t really tell us much. Schlaufman aims to change that.
His new definition makes sense because it relies on how and where these objects form, not simply their size. But not everyone will agree, of course.
Let the debate begin.
Regarding the Pluto controversy, it is disappointing that you portray the IAU view as fact and as something on which there is consensus among planetary scientists, when this is far from the case.
The IAU’s “third criterion” requiring an object to “clear the neighborhood of its orbit” is highly controversial and not accepted by many planetary scientists, who instead prefer to use the geophysical planet definition. According to that definition, a planet is any non-self-luminous spheroidal body orbiting a star, free floating in space, or even orbiting another planet. If an object is large enough and massive enough to be rounded by its own gravity, according to this definition, it is a planet.
In addition to the fact that the IAU definition was adopted by just four percent of its members, most of whom are not planetary scientists but other types of astronomers, and the fact that it was opposed in a formal petition by an equal number of professional planetary scientists, that definition has some serious flaws. Its greatest flaw is that it centers on an object’s location rather than on its intrinsic properties, as the determining factor on whether it is or is not a planet. If Earth were in Pluto’s orbit, it would not clear that orbit either. This means the definition could result in the same object being classed as a planet in one location and as not a planet in another location.
The problem could be easily corrected simply by recognizing dwarf planets as a subclass of planets, as intended by Alan Stern, the scientist who first coined the term “dwarf planet,” and whose term was misused by the four percent of the IAU who voted in 2006. Doing this would establish that some planets clear their orbits while others don’t. The latter are classed as dwarf planets.
The Pluto debate is not “getting tiresome.” The passage of time does not make a bad definition any better. What is getting tiresome is the lack of acknowledgment that there is an ongoing debate with two legitimate sides and that neither side should be given more primacy or legitimacy than the other one.
I was hoping you would post 🙂
The IAU definition is fact, for now, until it comes up again for debate/refinement.
It’s the language we need to use so that we all understand what we are talking about, across differing points of view and different languages. It’s a standard. We need a standard. For now, the IAU definition is the standard.
Standards can be changed with better information and/or sufficient protest from experts in the field. I fully suspect that the precedent of changing the definition will encourage reopening the subject for refinement in future meetings.
One more point: Should Earth really be considered the same type of object as Jupiter? The IAU view says yes. It puts Earth and Jupiter in the same category while excluding Pluto. Yet Earth has far more in common with Pluto than it does with Jupiter. Both Earth and Pluto are rocky worlds with solid surfaces. Both have nitrogen in their atmospheres; both are geologically layered into core, mantle, and crust; both have active geology; and both have large moons formed through giant impacts. In contrast, Jupiter’s has no known solid surface. Its composition is much like that of the Sun, mostly hydrogen and helium. It has its own “mini-solar system” of rings and moons.
Establishing planet as a broad category and then dividing the different types of planets into subcategories such as terrestrial, gas giant, ice giant, dwarf planet, super-Earth, hot-Jupiter, hot Neptune, etc., would successfully address this issue.
That seems to be a misrepresentation of what planet means. It is not a broad category that implies sameness between all bodies. To use your example, Earth and Jupiter are not considered the same type of object. Earth is a terrestrial planet while Jupiter is a gas giant. Pluto, meanwhile, is not a rocky body like Earth, its composed of a rocky core surrounded by a mantle of ice and water ice, and possibly a layer of liquid water at the core-mantle boundary. In that respect, it has more in common with Jupiter’s moons than it does with Earth.
I don’t think anyone considers Earth and Jupiter the same type of object. Adherents of the geophysical definition use the broad umbrella category of “planet” to include many subcategories–rocky planets, gaseous planets, dwarf planets, etc. Many also consider spherical moons to be satellite planets. That doesn’t mean they can’t be called moons; it’s just a way of distinguishing the moons large enough to be in hydrostatic equilibrium from those not large enough to attain that milestone. Maybe we should consider creating a new subcategory for worlds that have subsurface oceans; this would include Ceres, Europa, Ganymede, Enceladus, Titan, Triton, and Pluto.
But you claimed the IAU definition treats Earth and Jupiter as the same. And yes, I agree. These should be called “ocean worlds” since that has already entered the parlance.
But Runyon, Stern et.al. model accounts for moons too.. the IAU model too is flawed.. some of the IAU Planets dont really clear their orbits..
On a another note..
The size of the yet to verified (only postulated) celestial object (better to call Planet “nein”) is unknown, one could imagine it too maybe a Brown dwarf smaller than Jupiter..
That depends on what you mean by “clear their orbits”. If you are referring to NEOs and Jupiter’s Trojan and Greek population, that’s not the same. These populations of asteroids are dominated by the gravity of their respective planet, they do not share the same orbit. The same cannot be said about Ceres and the Asteroid Belt, or Pluto, Haumea, Makemake, Eris (etc) and the Kuiper Belt. They share their orbits with the many, many objects in these belts.
The obvious problem with this functional definition is what if a planet that forms by core accretion reaches 10x the size of Jupiter? Is this still a gas giant? The issue with the previous post is that if we drop the clearing orbit criterion from the definition of a planet then technically many moons are also planets. I thought dwarf planet was already a subclass of a planet functionally since it can’t clear it’s orbit due to the effects of a major planet nearby. If it gets pushed around by a larger planet then it is a dwarf planet.
Ganymede is a moon, even though it’s bigger than Mercury. Why? Because it’s orbit is not strictly heliocentric – it orbits Jupiter, which has a heliocentric orbit. If I recall correctly, that is one of the criteria for a moon-class celestial object – whether the object orbits another object besides the host star(s).
According to the study, the statistical results demonstrate that objects, larger than approx. 10x the mass of Jupiter, form around stars that have a metallicity below which rocky cores are not expected (insufficient metals to form any, according to a prevailing hypothesis). There are going to be some that are right on the line in terms of mass and in terms of the metallicity of the host star(s), and therefore, remote observation will not be sufficient to determine the most appropriate classification. That always happens in nature.
There are a lot of assumptions involved, however – we don’t know, for certain, whether Jupiter, or any of the other giants, have a rocky core. It’s an educated guess, based on a variety of direct and indirect observations of the planets’ properties. However, we really don’t know.