The Solar System is a really big place, and it takes forever to travel from world to world with traditional chemical rockets. But one technique, developed back in the 1960s might provide a way to dramatically shorten our travel times: nuclear rockets.
Of course, launching a rocket powered by radioactive material has its own risks as well. Should we attempt it?
Let’s say that you wanted to visit Mars using a chemical rocket. You would blast off from Earth and go into low Earth orbit. Then, at the right moment, you’d fire your rocket, raising your orbit from the Sun. The new elliptical trajectory you’re following intersects with Mars after eight months of flight.
This is known as Hohmann transfer, and it’s the most efficient way we know how to travel in space, using the least amount of propellant and the largest amount of payload. The problem of course, is the time it takes. Throughout the journey, astronauts will be consuming food, water, air, and be exposed to the long term radiation of deep space. Then a return mission doubles the need to resources and doubles the radiation load.
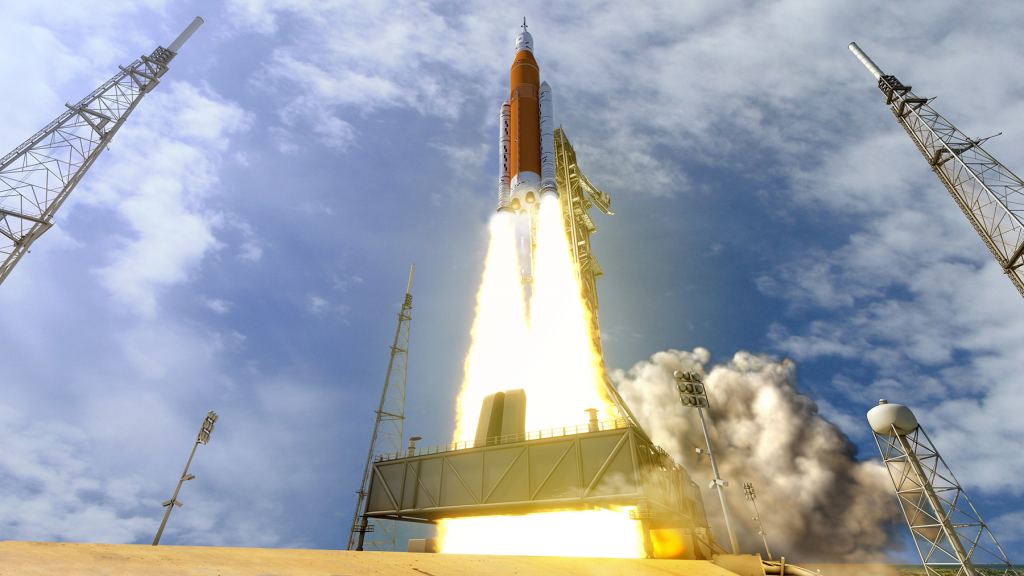
We need to go faster.
It turns out NASA has been thinking about what comes next after chemical rockets for almost 50 years.
Nuclear thermal rockets. They definitely speed up the journey, but they’re not without their own risks, which is why you haven’t seen them. But maybe their time is here.
In 1961, NASA and the Atomic Energy Commision worked together on the idea of nuclear thermal propulsion, or NTP. This was pioneered by Werner von Braun, who hoped that human missions would be flying to Mars in the 1980s, on the wings of nuclear rockets.
Well that didn’t happen. But they did perform some successful tests of nuclear thermal propulsion and demonstrated that it does work.
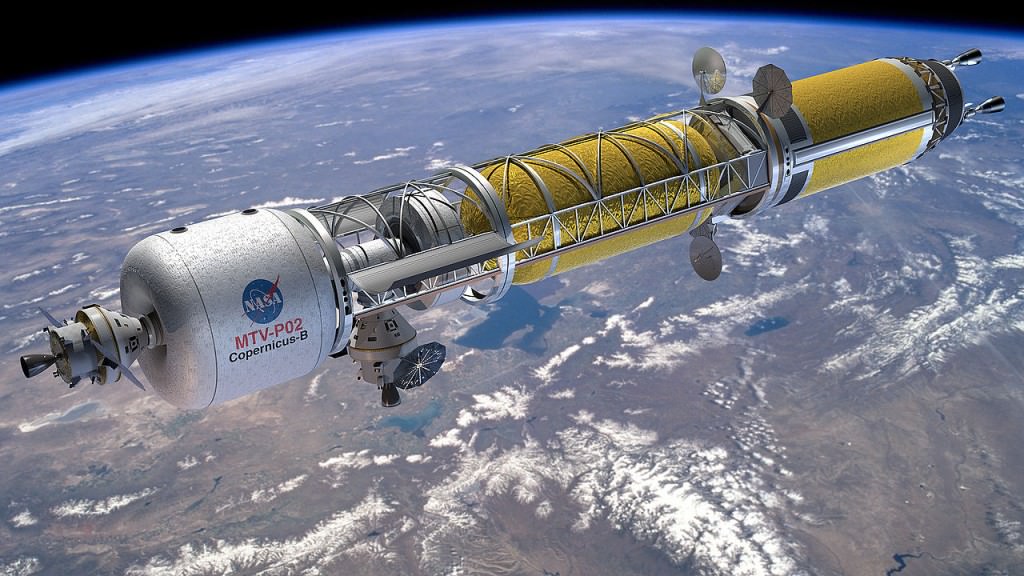
While a chemical rocket works by igniting some kind of flammable chemical and then forcing the exhaust gases out a nozzle. Thanks to good old Newton’s third law, you know, for every action there’s an equal and opposite reaction, the rocket receives a thrust in the opposite direction from the expelled gases.
A nuclear rocket works in a similar way. A marble-sized ball of Uranium fuel undergoes the process of fission, releasing a tremendous amount of heat. This heats up a hydrogen to almost 2,500 C which is then expelled out the back of the rocket at high velocity. Very very high velocity, giving the rocket two to three times the propulsion efficiency of a chemical rocket.
Remember the 8 months I mentioned for a chemical rocket? A nuclear thermal rocket could cut the transit time in half, maybe even 100 day trips to Mars. Which means less resources consumed by the astronauts, and a lower radiation load.
And there’s another big benefit. The thrust of a nuclear rocket could allow missions to go when Earth and Mars aren’t perfectly aligned. Right now if you miss your window, you have to wait another 2 years, but a nuclear rocket could give you the thrust to deal with flight delays.
The first tests of nuclear rockets started in 1955 with Project Rover at the Los Alamos Scientific Laboratory. The key development was miniaturizing the reactors enough to be able to put them on a rocket. Over the next few years, engineers built and tested more than a dozen reactors of different sizes and power outputs.
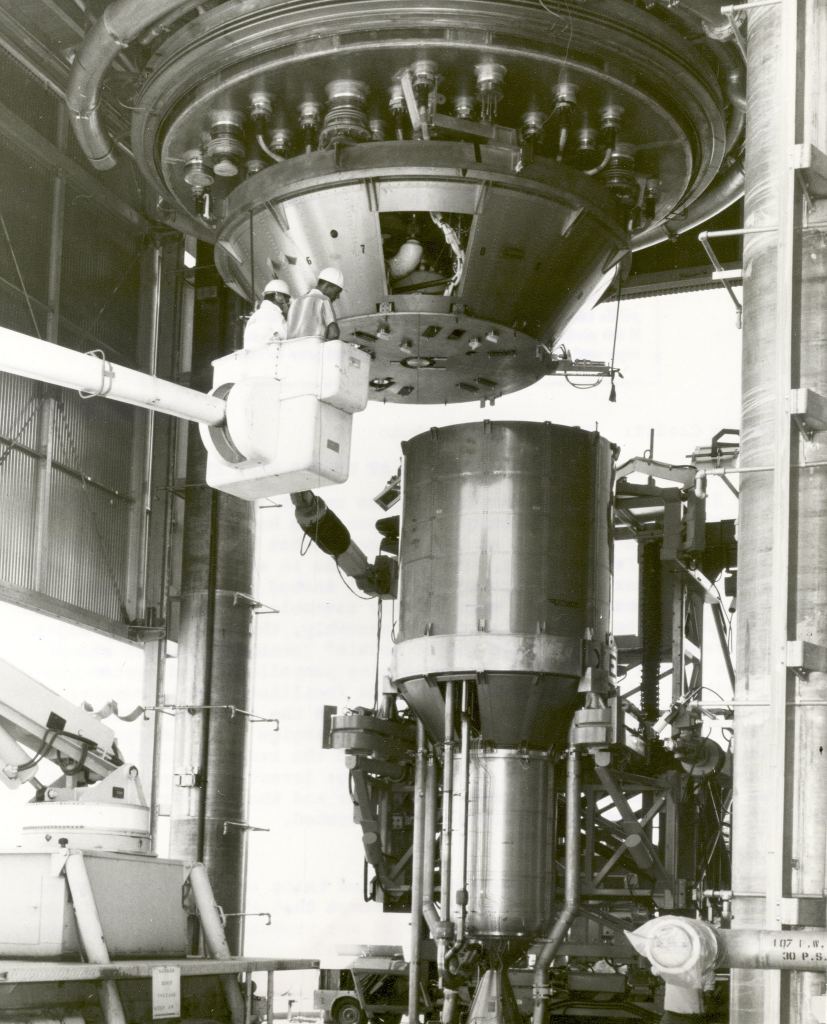
With the success of Project Rover, NASA set its sights on the human missions to Mars that would follow the Apollo landers on the Moon. Because of the distance and flight time, they decided nuclear rockets would be the key to making the missions more capable.
Nuclear rockets aren’t without their risks, of course. A reactor on board would be a small source of radiation to the astronaut crew on board, this would be outweighed by the decreased flight time. Deep space itself is an enormous radiation hazard, with the constant galactic cosmic radiation damaging astronaut DNA.
In the late 1960s, NASA set up the Nuclear Engine for Rocket Vehicle Application program, or NERVA, developing the technologies that would become the nuclear rockets that would take humans to Mars.

They tested larger, more powerful nuclear rockets, in the Nevada desert, venting the high velocity hydrogen gas right into the atmosphere. Environmental laws were much less strict back then.
The first NERVA NRX was eventually tested for nearly two hours, with 28 minutes at full power. And a second engine was started up 28 times and ran for 115 minutes.
By the end, they tested the most powerful nuclear reactor ever built, the Phoebus-2A reactor, capable of generating 4,000 megawatts of power. Thrusting for 12 minutes.
Although the various components were never actually assembled into a flight-ready rocket, engineers were satisfied that a nuclear rocket would meet the needs of a flight to Mars.
But then, the US decided it didn’t want to go to Mars any more. They wanted the space shuttle instead.
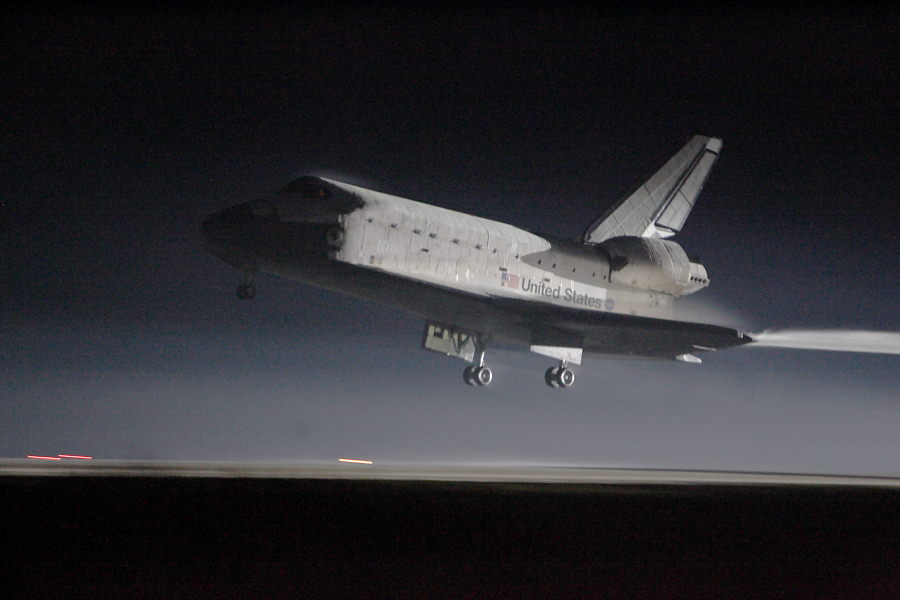
The program was shut down in 1973, and nobody tested nuclear rockets since then.
But recent advances in technology have made nuclear thermal propulsion more appealing. Back in the 1960s, the only fuel source they could use was highly enriched uranium. But now engineers think they can get by with low-enriched uranium.
This would be safer to work with, and would allow more rocket facilities to run tests. It would also be easier to capture the radioactive particles in the exhaust and properly dispose of them. That would bring the overall costs of working with the technology down.
On May 22, 2019, US Congress approved $125 million dollars in funding for the development of nuclear thermal propulsion rockets. Although this program doesn’t have any role to play in NASA’s Artemis 2024 return to the Moon, it – quote – “calls upon NASA to develop a multi-year plan that enables a nuclear thermal propulsion demonstration including the timeline associated with the space demonstration and a description of future missions and propulsion and power systems enabled by this capability.”
Nuclear fission is one way to harness the power of the atom. Of course, it requires enriched uranium and generates toxic radioactive waste. What about fusion? Where atoms of hydrogen are squeezed into helium, releasing energy?

The Sun has fusion worked out, thanks to its enormous mass and core temperature, but sustainable, energy positive fusion has been elusive by us puny humans.
Huge experiments like ITER in Europe are hoping to sustain fusion energy within the next decade or so. After that, you can imagine fusion reactors getting miniaturized to the point that they can serve the same role as a fission reactor in a nuclear rocket. But even if you can’t get fusion reactors to the point that they’re net energy positive, they can still provide tremendous acceleration for the amount of mass.
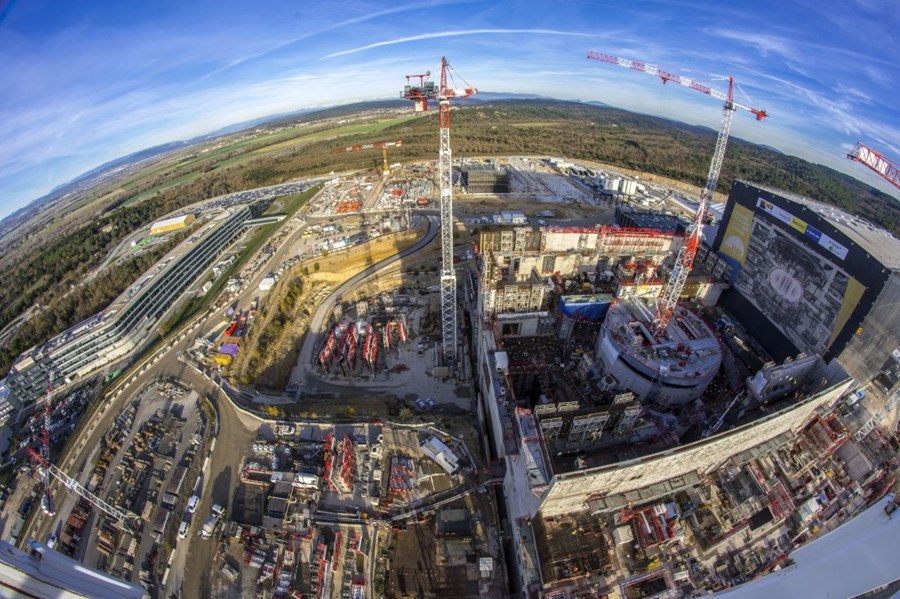
And maybe we don’t need to wait decades. A research group at the Princeton Plasma Physics Laboratory is working on a concept called the Direct Fusion Drive, which they think could be ready much sooner.
It’s based on the Princeton Field-Reversed Configuration fusion reactor developed in 2002 by Samuel Cohen. Hot plasma of helium-3 and deuterium are contained in a magnetic container. Helium-3 is rare on Earth, and valuable because fusion reactions with it won’t generate the same amount of dangerous radiation or nuclear waste as other fusion or fission reactors.
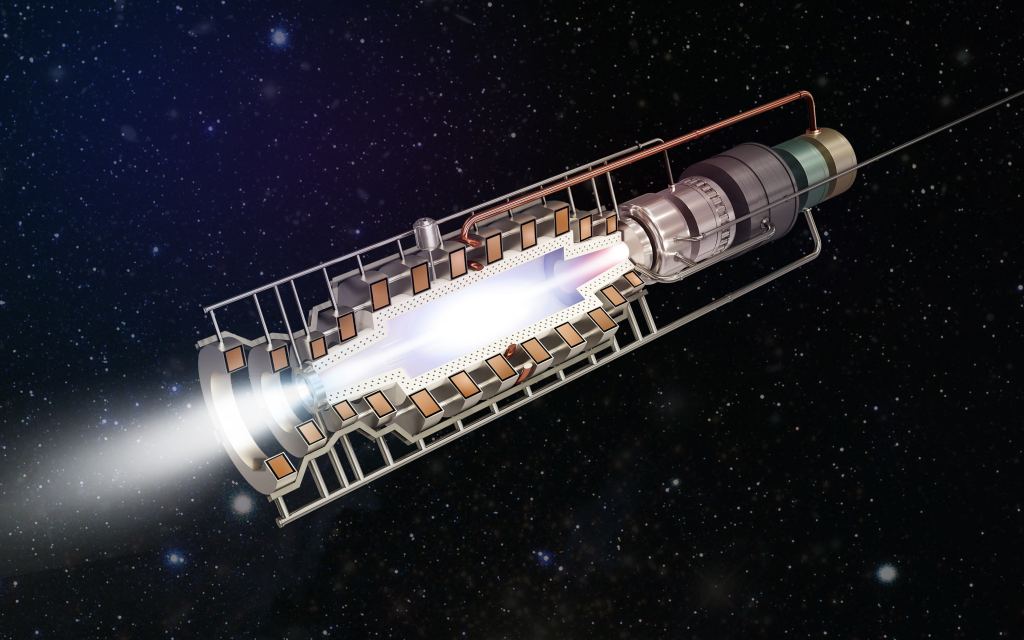
As with the fission rocket, a fusion rocket heats up a propellant to high temperatures and then blasts it out the back, producing thrust.
It works by lining up a bunch of linear magnets that contain and spin very hot plasma. Antennae around the plasma are tuned to the specific frequency of the ions, and create a current in the plasma. Their energy gets pumped up to the point that the atoms fuse, releasing new particles. These particles wander through the containment field until they’re captured by the magnetic field lines and they get accelerated out the back of the rocket.
In theory, a fusion rocket would be capable of providing 2.5 to 5 Newtons of thrust per megawatt, with a specific impulse of 10,000 seconds – remember 850 from fission rockets, and 450 from chemical rockets. It would also be generating electricity needed by the spacecraft far from the Sun, where solar panels aren’t very efficient.
A Direct Fusion Drive would be capable of carrying a 10 tonne mission to Saturn in just 2 years, or a 1-tonne spacecraft from Earth to Pluto in about 4 years. New Horizons needed almost 10.
Since it’s also a 1 megawatt fusion reactor, it would also provide power for all the spacecraft’s instruments when it arrives. Much much more than the nuclear batteries currently carried by deep space missions like Voyager and New Horizons.
Imagine what kinds of interstellar missions might be on the table with this technology too.
And Princeton Satellite Systems isn’t the only group working on systems like this. Applied Fusion Systems have applied for a patent for a nuclear fusion engine that could provide thrust to spacecraft.
I know it’s been decades since NASA seriously tested nuclear rockets as a way to shorten flight times, but it looks like the technology is back. Over the next few years I expect to see new hardware, and new tests of nuclear thermal propulsion systems. And I am incredibly excited at the possibility of actual fusion drives taking us to other worlds. As always, stay tuned, I’ll let you know when one actually flies.