When the International Space Station (ISS) runs low on basic supplies – like food, water, and other necessities – they can be resupplied from Earth in a matter of hours. But when astronauts go the Moon for extended periods of time in the coming years, resupply missions will take much longer to get there. The same holds true for Mars, which can take months to get there while also being far more expensive.
It’s little wonder then why NASA and other space agencies are looking to develop methods and technologies that will ensure that their astronauts have a degree of self-sufficiency. According to NASA-supported research conducted by Daniel Tompkins of Grow Mars and Anthony Muscatello (formerly of the NASA Kennedy Space Center), ISRU methods will benefit immensely from some input from nature.
Tompkins and Dr. Muscatello began their collaborative relationship in 2018 shortly after the latter retired from NASA KSC, where he spent the previous two decades working on chemical systems for In-Situ Resource Utilization (ISRU) applications. Later that year, Muscatello delivered a speech at the annual Mars Society Convention on the subject of how Mars could be terraformed using oxygen and self-replicating robots.
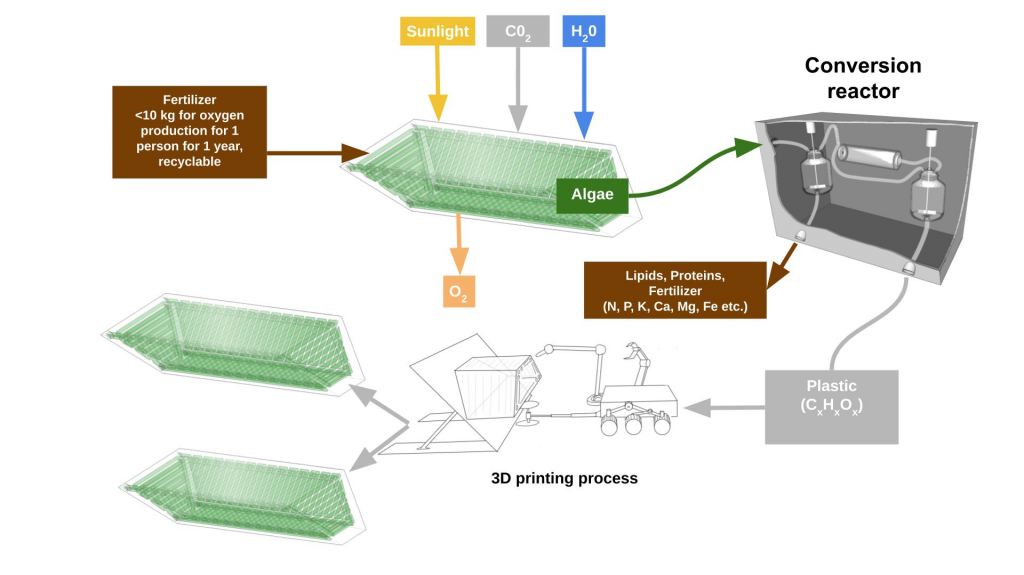
Shortly thereafter, Tompkins contacted Dr. Muscatello and the two began collaborating on a project designed to improve conventional ISRU techniques. With the support of researchers from NASA KSC, the NASA Jet Propulsion Laboratory, the Moon Village Association, and The Bionetics Corporation, Tompkins and Muscatello produced a joint study describing their approach.
This study was presented at the tenth joint meeting of the Planetary & Terrestrial Mining Sciences Symposium (June 11th-14th, 2019), which coinciding with the 20th anniversary of the Space Resources Roundtable (SRR). Here, the pair described how traditional ISRU techniques used to establish a lunar base could be vastly improved by incorporating growing and self-replicating living organisms (aka. the Green Moon concept).
As Dr. Muscatello described it to Universe Today via email:
“GrowMars is based on biological processes to make oxygen from CO2 with chemical processing of the algae to convert it to the starting products to make polymers for structural applications. Conventional ISRU is based on physical and chemical processes only.”
The pair detailed this concept further in a peer-reviewed study that addressed how these same biological and agricultural methods could be used to create vast greenhouses on Mars (aka. GrowMars). In this process, algae is grown in greenhouses that use locally-harvested water, atmospheric CO2 (on Mars), waste CO2 from human respiration and waste, or CO2 and carbon monoxide (CO) from lunar cold traps, and sunlight.
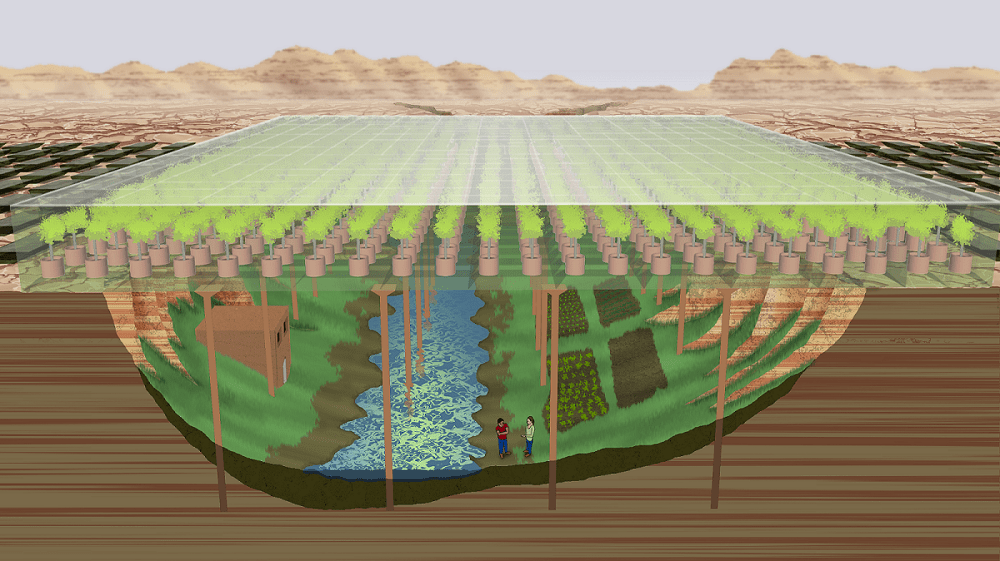
This biomass, which is rich in carbohydrates, is then fed into a conversion reactor to produce polyethylene furandicarboxylate (PEF), a bioplastic derived from carbohydrates (C8H6O). This bioplastic can be used as a bonding agent that is then combined with lunar or Martian regolith to create a polymer composite concrete, which in turn is used as feedstock for robotic 3D printers to create more greenhouses.
According to their greenhouse design, the composite building material is printed out to make transparent blocks that are rectangular (lengthwise) and trapezoidal (crosswise). This shape optimizes the absorption of solar energy by ensuring that the greenhouses can constantly be tilted towards the Sun and allows for the blocks to be tessellated (stacked with no space between them) to form larger structures.
As Tompkins explained the process to Universe Today via email:
“Biomass to plastic conversion is well studied and practiced in an effort to make sustainable and petroleum independent plastics. Basically, the carbohydrate portion of biomass is processed into clear plastics at a high rate with low power and mass required. [The] key thing to note [is that] not all bioderived plastics are biodegradable.”
In short, this bioplastic can be generated using sugars (rather than petrochemicals) and is durable enough for off-world use. Between that and the shape they are printed in, the blocks can be combined to create many different types of structures. This includes homes and parks for human settlers as well as additional greenhouses.
Over time, these new greenhouses could grow more algae to create more blocks, thus “growing” a colony over time. When placed over natural depressions, like craters or extended valleys (aka. fossae, which are common on Mars), they can work as both greenhouses and radiation shields. In fact, the ability to shield against harmful radiation is one of the most beneficial aspects of this system.
Traditionally, radiation shielding consists of metal panels (like lead) since they have a good weight-to-protection ratio. Whereas barriers made of other materials can also block cosmic and solar rays, they typically have to be very thick to do so. Unfortunately, metal panels do not protect crews from the secondary particles that result from collisions, which create secondary particles that will “shower” crews.
By comparison, water and plastic have been shown to be effective at blocking radiation while generating no secondary particles – the same holds true of bioplastics. The only impediment is, natural barriers need to be up to 3 m (~10 ft) thick in order to reliably protect against solar and cosmic rays. But with a tessellated structure that also acts as a dome, a colony would have all the natural shielding it requires.
In addition, the conversion reactor also creates useful byproducts, like proteins, lipids (fat cells), and minerals that can be used in the manufacture of chemical fertilizers (nitrogen, phosphorous, potassium, calcium, magnesium, iron, etc.) On top of that, says Tompkins, they can be used to manufacture other crucial resources like food, oxygen, biofuel, rocket fuel – the list goes on.
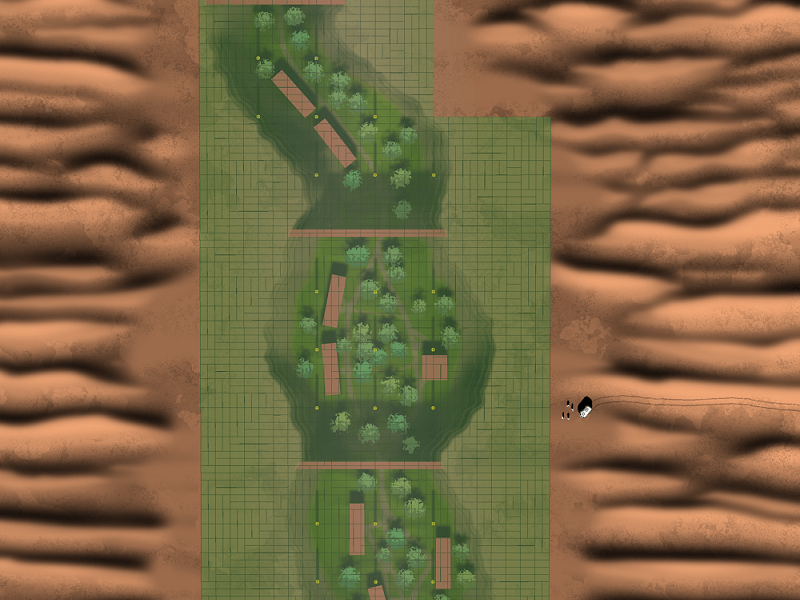
As Dr. Muscatello summarized the process:
“GrowMars generates more of the polymer blocks in which the algae grows, thereby expanding the oxygen and polymer production capacity exponentially with time, up to the limits of the algae-to-polymer process units, which can be over-sized in anticipation of this aspect if desired. The algae replicates itself as long as CO2, water, and a small amount of fertilizer are provided.
“Conventional ISRU is always limited to the capacities of the as-built systems, i.e. to get more production, more units are required. Thus, the algae-based system could have mass and power advantages over conventional ISRU. The algae based systems can also be configured to provide radiation shielding with the water and polymer blocks.”
When it comes to Project Artemis and NASA’s long-term vision of creating a program of “sustainable lunar exploration,” one of the principal focuses is on how local resources can be leveraged to provide self-sufficiency. The same holds true for mission architectures to Mars and the outer Solar System. In all cases, the plan is to harvest water ice, regolith, and other local resources so that resupply missions can be few and far between.
Traditional ISRU techniques are focused primarily on harnessing locally-available resources, like water ice and regolith. In contrast, this new approach focuses on how harnessing local water and minerals, as well as energy from the Sun, and carbon dioxide and carbon monoxide from human waste, to multiply local resource potential.
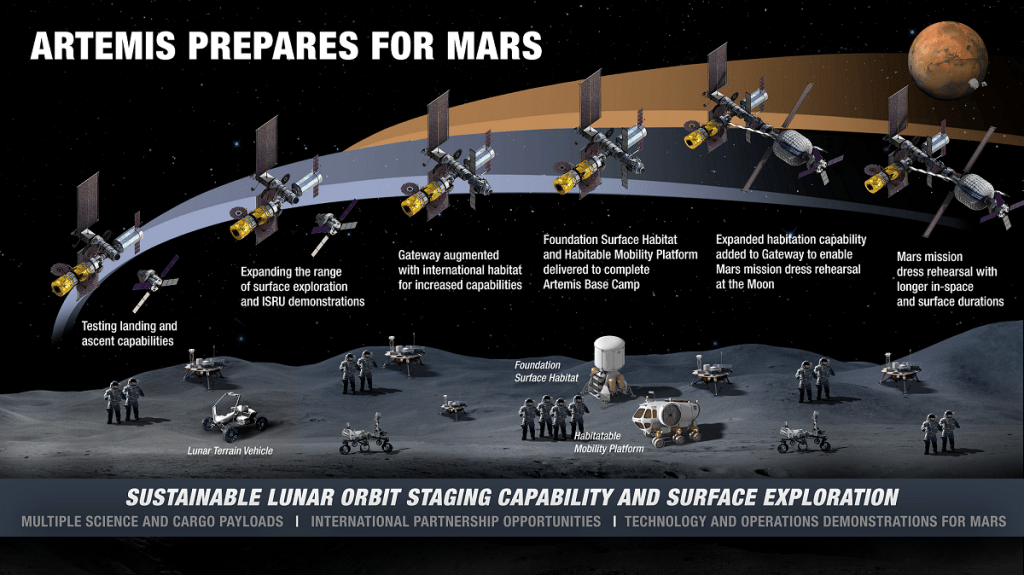
As Tompkins put it, this technique would ensure the production of more food and better building materials:
“We are leveraging these efforts in the space context with unique functionality of being carbon negative, (producing net oxygen), enabling more photosynthesis area, radiation protection, building material, all in an expanding loop system that is biologically and functionally integrated. All with the hope of self-sufficiency and outpacing entropy at a basic needs level.”
“Bio-based systems have the potential to reduce the mass and power required to provide oxygen and polymers while having the ability to expand their production rates, thus reducing the costs of launch mass, the volume launched, and power requirements,” added Dr. Muscatello. “Such advantages can make exploration and settlement less expensive and faster.”
Many of the ideas presented by Tompkins and Muscatello were also touched on by Dr. Robert Zubrin in a recent study that appeared on Centauri Dreams – titled “Sublake Settlements for Mars.” In addition to being an aerospace engineer and the founder of the Mars Society, Dr. Zubrin is also a noted advocate for the exploration, colonization, and terraforming of Mars.
In this essay, Zubrin presents a variation on the paraterraforming concept, where sections of a planet are enclosed and ecologically engineered to be habitable. Specifically, he explored how settlements could be built within ice-filled craters on Mars, where the lower layers of ice could be melted to create a subglacial lake. Over time, the presence of warm water and organic molecules could allow for the formation of a biosphere.
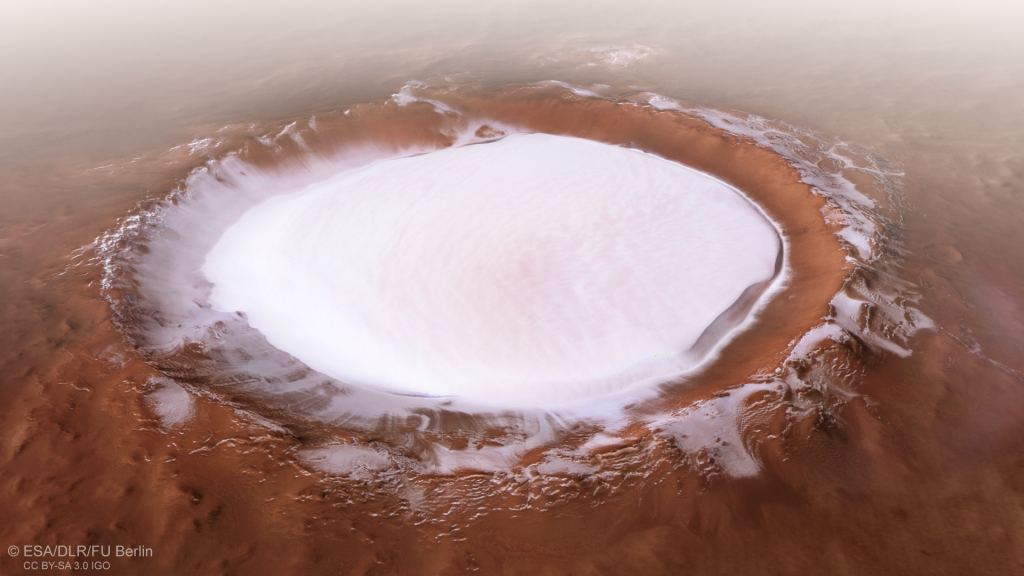
Housing created within the lake would benefit from the fact that the external pressure would be similar to Earth’s atmosphere (100 kPa, or 1 bar). This is preferable in many ways to habitats built on the surface, where atmospheric pressure is (on average) about 0.5% what we experience here on Earth. On top of that, the thick ice and deep water would provide all the necessary radiation shielding for a settlement to survive.
Taken as a while, Tompkins and Muscatello’s concept for “growing” settlement on the Moon and Mars represents a practical and scalable concept for off-world colonies. But perhaps some of the most exciting implications of this study is the impact it could have here on Earth. As Tompkins reminds us, the innovation that resulted from the Apollo Program resulted in countless applications here at home.
These applications were made possible thanks to NASA’s Technology Transfer Program and have been cataloged since 1976 by NASA Spinoff. Whereas the Apollo Program resulted in commercial, medical, and industrial applications ranging from microwave ovens, heart monitors, and MRIs to GPS and Lidar. This time, NASA’s goals call for innovations that can ensure self-sufficiency, sustainability, and cost-effectiveness.
Based on current UN projections, Earth’s population is expected to peak at 10 billion by the year 2050. Simultaneously, climate change will continue to disrupt the very systems humans depend upon for their very livelihood and survival. The only way to prevent the worst from happening (widespread famine, economic collapse, mass migrations, etc.) is to employ technologies that allow us to do more with less.
It’s not hard to see how technologies that can leverage biomass to create petroleum-free plastics (as well as fertilizers and food) will be a boon for future generations. There are also parallels between this technology and the work of Stanford Professor Steven Chu, former Energy Secretary for the Obama administration and the man who proposed the “Glucose Economy,” an alternative to the current petroleum-based economy.
In the near future, Tompkins and Muscatello are hoping to secure additional funding so they can mount a full-cycle demonstration of the technology. If all goes well, this type of biomass-to-bioplastics and farming method could become a regular feature of lunar and Martian colonies. It could even become a regular feature of life here on Earth.
As always, our efforts to send humans into space are yielding tangible benefits here at home. And when the technology designed to address what is needed to survive out there also addresses some of our greatest needs down here, those benefits can be enormous.
Further Reading: GrowMars, Space Resources Roundtable study, Peer-reviewed study, Centauri Dreams
There is a know problem on Mars, the relatively frequent dust storms.
They can cover quite significant parts of the planet and could potentially stop all photosyntesis processes.
For others like me, who are among the lesser cognoscenti of NASA, ISRU stands for In Situ Resource Utilization and has it’s own page on nasa.gov: https://isru.nasa.gov/
Think ‘farming, mining, refining, and manufacturing on other planets, moons, asteroids, space stations, etc….
Yes, we are aware of what ISRU is. Was that not clear in the article?
No. I am afraid it was not.
ISRU is first mentioned in the last line of the second paragraph. It is then used seven more times in the body of the article. At no point is the acronym defined.
Even though I consider myself a moderate space/rocketry/astronomy enthusiast, I do not recall having ever heard or read ISRU before. I actually did a search on ISRU after the fourth mention so I could better understand the article.
I admit that there are many people, yourself probably included, who are FAR more knowledgeable than I on all things NASA. My comment was (hopefully) a gentle reminder that many of us will becoming across these terms for the first time and so a brief spelling out of an acronym (maybe with a link) will be greatly appreciated!
Good article:). I’m curious as to whether carbon is widespread enough on Luna to support this approach.
As to the discussion in the comments section:
In any article intended to be read by a mass audience it’s important to define initialisms on their first use.
It’s good to remember that people are being directed to UT from places like Google News, not just from specialty forums or low volume Reddit subs. I know what ISRU means because it’s a special interest of mine, but it’s a *very* uncommon initialism that most people will never have encountered.
I actually once had someone (an intelligent, knowledgeable person) call me out as elitist for using the term “in situ resource utilization”, because I could have just used English instead. Once I figured out what they meant (because that *is* English from my point of view) I had to explain to them that I wasn’t just hauling out Latin to sound smarter; it is an actual term used by both industry professionals and academics. It so rarely used outside of our particular circle of interest that they’d never heard the phrase itself before, never mind the initialism.