Lately there has been a flood of interest in gravitational waves. After the first official detection at LIGO / Virgo in 2015, data has been coming in showing how common these once theoretical phenomena actually are. Usually they are caused by unimaginably violent events, such as a merging pair of black holes. Such events also have a tendency to emit another type of phenomena – light. So far it has been difficult to observe any optical associated with these gravitational-wave emitting events. But a team of researchers hope to change that with the full implementation of the Gravitation-wave Optical Transient Observer (GOTO) telescope.
The GOTO project is designed specifically to find and monitor the parts of the sky that other instruments, such as LIGO, detect gravitational waves from. Its original incarnation, known as the GOTO-4 Prototype, was brought online in 2017. Located in La Palma, in the Canary Islands, this prototype consisted of four “unit telescopes” (UTs) housed in an 18ft clamshell dome. In 2020, this prototype was upgraded to 8 UTs, allowing for a much wider view of the sky.
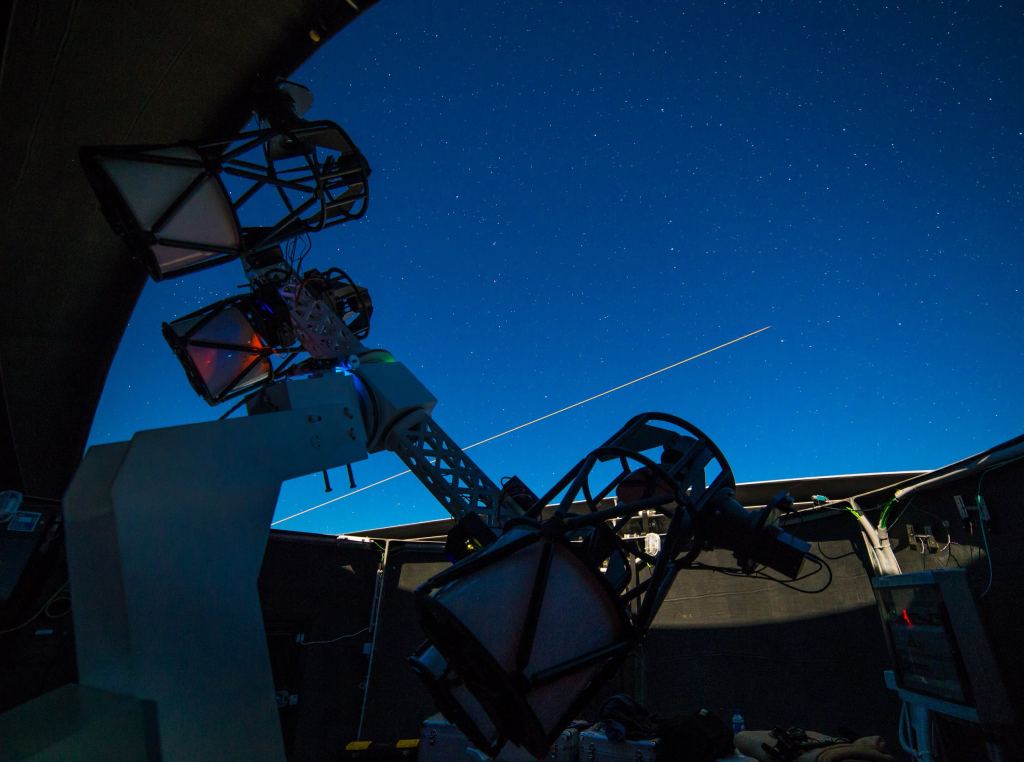
Credit: GOTO Project
The wide field of view is necessary for its work detecting gravitational-wave based optical phenomena, as directionality of gravitational waves are notoriously difficult to pin down. The wider the field of view of a telescope, the more likely it will be able to detect an event that happens.
As such, the operators of GOTO started an upgrade plan in 2020. These upgrades included an additional 8 UT in a separate dome at the same observatory, which is due to be added in early 2021. More ambitiously, the team plans to recreate the two-unit array in La Palma at the Siding Spring Observatory in New South Wales, Australia. With these telescopes on opposite sides of the world, GOTO will “enable close to 24-hour observations, ensuring that GOTO is able to react to alerts whenever they occur” according to a recent paper.
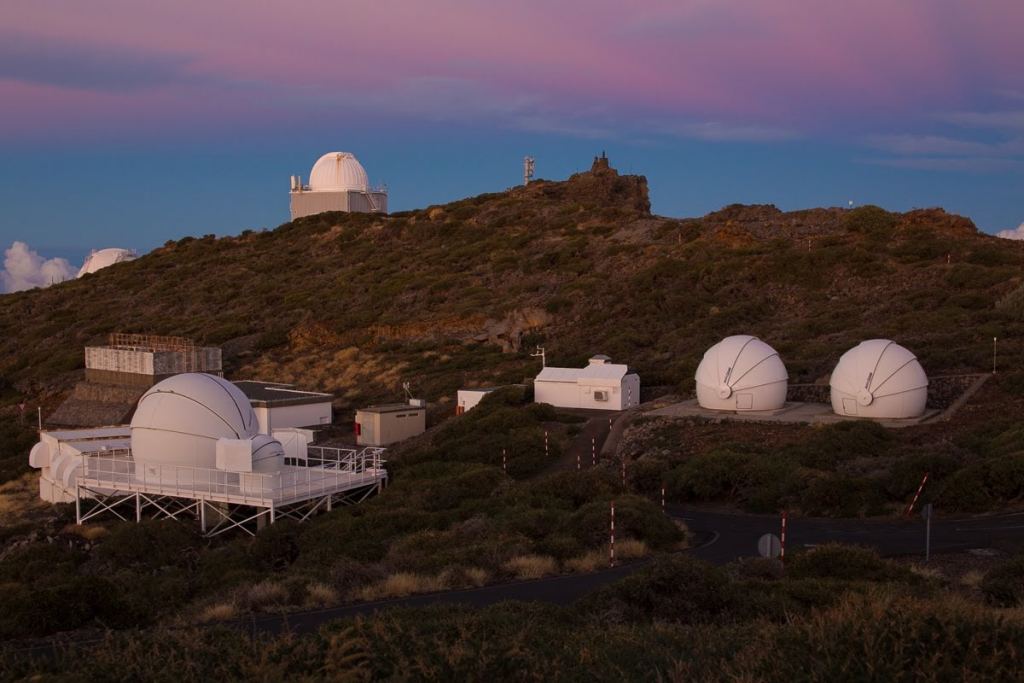
Credit: GOTO Project
Those alerts are an extremely important part of GOTO’s observational planning. They come from NASA’s Gamma-ray Coordination Network (GCN), an alert system that monitors not only gravitational waves, but also other phenomena that could produce interesting optical data, such as kilonovas or gamma ray bursts.
GOTO monitors this network through it’s software package, which is also a key component to overall system operation. The GOTO Telescope Control System (G-TeCS) is a custom written Python script that monitors for signals of interest, calculates which signal is the highest priority, and then physically moves the telescopes to an observing position. It is also able to do all of that in less than 30 seconds, allowing for an extremely quick turnaround in order to observe these transient phenomena of interest.
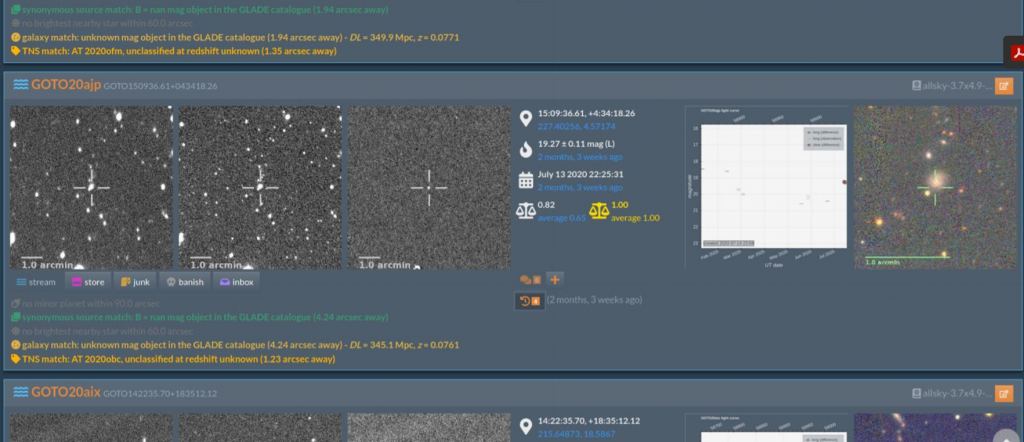
Credit: GOTO Project
Once the telescopes are positioned, G-TeCS is also able to collect and analyze images. It compares any images it captures with a calibration image, and uses a type of artificial intelligence known as a “convolutional neural network” to assign a score to the likelihood that it detected a signal of interest. As with so much AI assisted research, humans are the last part in the analysis chain. Researchers use a tool called GOTO Marshall to individually validate high interest targets, and can also schedule follow-up observations with other telescopes in the area.
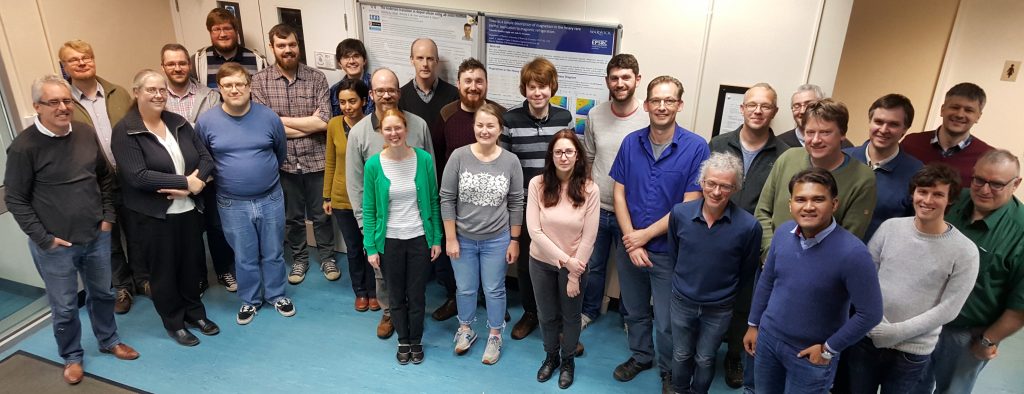
Credit: GOTO Project
All of this software system is controlled remotely at the University of Warwick, who leads the GOTO project, which includes 9 other institutions from the UK, Australia, Thailand, Spain, and Finland. As they continue to implement their planned improvements, and data continues to come in, we’ll start to be able to visualize the catastrophic events associated with some of the most violent phenomena in the universe.
Learn More:
arXiv: The Gravitational-wave Optical Transient Observer (GOTO)
University of Warwick: Gravitational wave Optical Transient Observatory
Lead Image Credit: The prototype GOTO telescope. Credit: GOTO Project
To underscore how important these new events are, already the first one has ushered in an independent method to observe the universe expansion rate. A new paper synthesized information from various LIGO, kilonova and pulsar studies to improve on that.
“We performed a joint analysis of the gravitational-wave event GW170817 with its electromagnetic counterparts AT2017gfo and GRB170817A, and the gravitational-wave event GW190425, both originating from neutron-star mergers. We combined these with previous measurements of pulsars using x-ray and radio observations, and nuclear-theory computations using chiral effective field theory, to constrain the neutron-star equation of state. We found that the radius of a 1.4–solar mass neutron star is 11.75 +0.86 -0.81 km at 90% confidence and the Hubble constant is 66.2 +4.4 -4.2 at 1sigma uncertainty.”
“We found that the radio inclination measurement reduces the existing uncertainty on the Hubble constant by more than the kilonova measurement, at least for this single event.The uncertainty does not allow us to resolve the tension between measurements through type-Ia supernovae (30) and the Planck measurement of the cosmic microwave background(31), but our results indicate a preference forthe latter (Fig. 2B)”
[“Multimessenger constraints on the neutron-star equation of state and the Hubble constant”, Dietrich et al., Science, 18 December 2020]