As we continue to search for dark matter particles, one thing is very clear: they cannot be any of the elementary particles we’ve discovered so far. The particles would need to have mass, but interact with light only weakly. Of the known particles, neutrinos fit that description, but neutrinos have a tiny mass, and aren’t nearly enough to explain dark matter. Some other kind of particle must make up the majority of dark matter.
Physicists have looked beyond the standard model of particle physics for possible candidates, and one that has looked promising is known as the axion. Axions were first proposed in the 1970s to deal with a problem of symmetry in particle physics. As Emmy Noether first pointed out, symmetry is fundamental to the nature of physics. In particle physics, there are three important symmetries: charge, parity, and time.
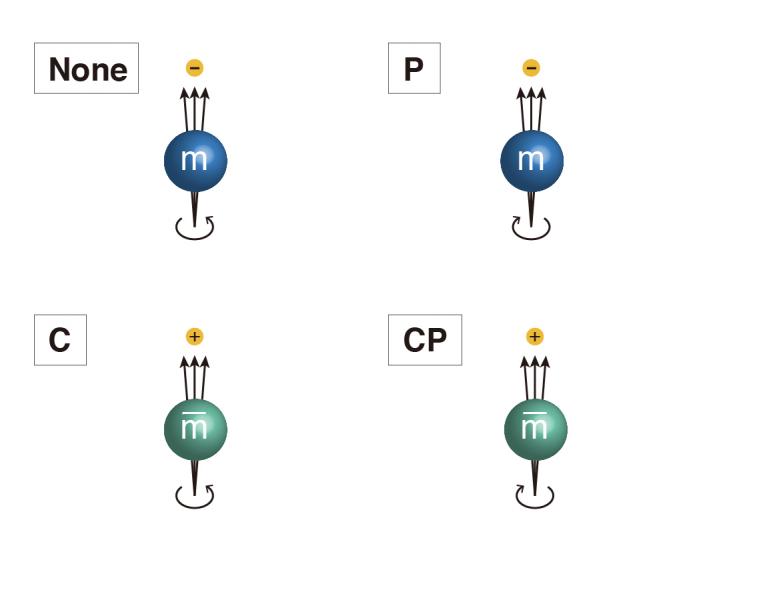
Time symmetry deals with how interactions look the same forwards and backward in time. Imagine two billiard balls colliding on a pool table. If you saw a video of the collision, it would be difficult to determine whether the video was reversed without some other clue. Billiard balls obey time symmetry. Charge symmetry deals with how charges interact. Two positive charges repel each other, but so do two negative charges. So if you see two charged particles push away from each other, you only know they have the same type of charge. Parity has to deal with mirror-image interactions. If a moon orbits a planet, it would behave the same way whether it’s orbiting clockwise or counterclockwise. These symmetries can also be combined. For example, an interaction that is symmetrical under a reversal of both charge and parity would obey CP-symmetry.
It turns out that these symmetries aren’t perfect. The weak interaction is known to violate CP-symmetry in certain interactions about one time out of every thousand. The standard model predicts this, but it also predicts there should be a similar violation in the strong force. Physicists have looked for such a violation, but haven’t found one. This is where the axion comes in. If axions exist, they would act to suppress CP-violation in the strong force, solving the symmetry problem in the standard model. Theoretically, axions should be abundant, have mass and no charge, thus making them a good candidate for dark matter particles. And a recent study has tried to find them.
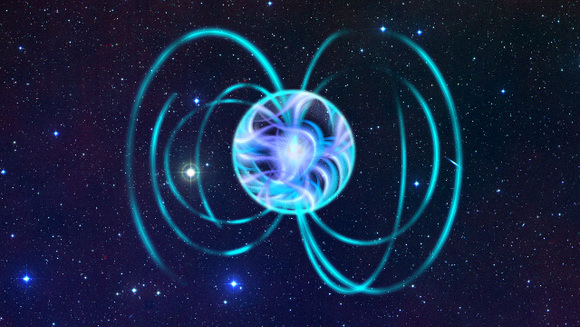
Since axions have no charge, they normally wouldn’t interact with light at all. But if they are in an intense magnetic field, they can be triggered to emit photons. If enough axions are clustered together, these photons should create a detectable radio signal. So the team looked for these signals coming from neutron stars. Neutron stars are known to have intense magnetic fields, and with their stellar mass and high density, they would likely attract plenty of axions.
Using data from the Green Bank Telescope in West Virginia and the Effelsberg Telescope in southwest Germany, the team looked at radio signals from two neutron stars. They found no axion signal in either. This actually puts a damper on the idea that axions exist, though it doesn’t completely rule out axions.
So it looks like both physicists and astronomers have reason to be disappointed. Physicists likely have to look elsewhere for a solution to their symmetry problem, and astronomers will continue to look for dark matter particles.
Reference: Foster, Joshua W., et al. “Green Bank and Effelsberg Radio Telescope Searches for Axion Dark Matter Conversion in Neutron Star Magnetospheres.” Physical Review Letters 125.17 (2020): 171301.