The search for planets beyond our Solar System (extrasolar planets) has grown by leaps and bounds in the past decade. A total of 4,514 exoplanets have been confirmed in 3,346 planetary systems, with another 7,721 candidates awaiting confirmation. At present, astrobiologists are largely focused on the “low hanging fruit” approach of looking for exoplanets that are similar in size, mass, and atmospheric composition to Earth (aka. “Earth-like.”)
However, astrobiologists are also interested in finding examples of “exotic life,” the kind that emerged under conditions that are not “Earth-like.” For example, a team of astronomers from the University of Cambridge recently conducted a study that showed how life could emerge on ocean-covered planets with hydrogen-rich atmospheres (aka. “Hycean” planets). These findings could have significant implications for exoplanet studies and the field of astrobiology.
The research was led by Dr. Nikku Madhusudhan, a Reader in Astrophysics and Exoplanetary Science from the University of Cambridge’s Institute of Astronomy (IoA). He was joined by Ph.D. astrophysics student Anjali Piette (Dr. Madhusudhan is her supervisor) and fellow IoA member Dr. Savvas Constantinou. The study that describes their findings, titled “Habitability and Biosignatures of Hycean Worlds,” recently appeared in The Astrophysical Journal.

Life on Little Ice Giants?
Of all the exoplanets that have been discovered in the past 30 years, the vast majority have either been predominantly rocky planets several times the mass of Earth (“Super-Earths”) or ice giants with hydrogen-rich atmospheres (“mini-Neptunes”) or somewhere in between. Whereas Super-Earths account for about 30% (1,383) of all exoplanets discovered to date, mini-Neptunes are the most plentiful, accounting for 34% (1,531).
Most mini-Neptunes are over 1.7 and 3.9 times the size of Earth and are believed to have interiors composed of ice, rock, and oceans of volatile elements. Previous studies of such planets have found that the pressure and temperature conditions beneath their hydrogen-rich atmospheres would be too great to support life. However, in a previous study, Nikku Madhusudhan and his team found that these planets could support life under certain conditions.
In particular, they examined the exoplanet K2-18b, a mini-Neptune that was the focal point of a lot of attention in 2019 when two different teams reported detecting water vapor in its hydrogen-rich atmosphere. The results Dr. Madhusudhan and his team obtained from this study led them to investigate the full range of planetary and stellar properties that would allow mini-Neptunes to be potentially habitable.
This led them to identify a new class of planets that they named “Hycean,” which is a portmanteau of the words “hydrogen” and “ocean.” Like so-called “water worlds,” Hycean worlds would be covered in planet-wide oceans but would have atmospheres dominated by hydrogen (consistent with gas giants). The presence of this atmospheric hydrogen would allow for a greenhouse effect, which would help ensure that the surface oceans remain liquid.
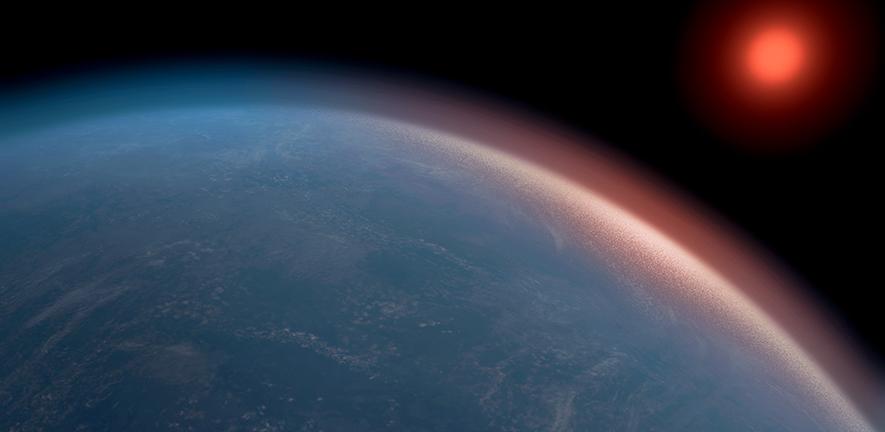
Characteristics
Planets that fall into this classification would have a diameter about 2.6 times that of Earth and have atmospheric temperatures of up to 200°C (392°F), depending on the nature of their host star and the planet’s proximity to it. This is similar to what scientists think conditions were like on Earth billions of years ago, at a time when the first single-celled microbial organisms began to emerge.
As Dr. Madhusudhan summarized in a recent University of Cambridge news release, these results could mean that there is a class of exoplanets more plentiful than “Earth-like” ones that support life, making it that much easier for astrobiologists to find:
“Hycean planets open a whole new avenue in our search for life elsewhere. Essentially, when we’ve been looking for these various molecular signatures, we have been focusing on planets similar to Earth, which is a reasonable place to start. But we think Hycean planets offer a better chance of finding several trace biosignatures.”
In addition, the team also identified several prime Hycean candidates for follow-up observations in their study. Many of these are larger and hotter than Earth but can still be covered in large oceans with the types of conditions that could support microbial life. This life would likely be concentrated in extreme environments, like hydrothermal at the ocean-mantle boundary, similar to what has been observed here on Earth.
This type of exoplanet might include a subclass of tidally-locked “dark Hycean” planets, where habitable conditions exist only on their permanent night sides. The side facing the planet’s host star would be too hot to maintain water in liquid form indefinitely and transfer heat to the dark side through oceanic and atmospheric convection. There’s also the possibility of “cold Hycean” worlds that receive little radiation from their stars and have icy shells.

Implications for Astrobiology
Planets of this size are the most common among the known exoplanet population, though they have not been studied nearly as much detail as super-Earths. But their commonality alone means that some of the most promising places to look for life elsewhere in the Galaxy may have been hiding in plain sight. In addition, these planets allow for a much wider circumsolar habitable zone than Earth-like planets.
Locating these exoplanets among the statistically significant Super-Earth and mini-Neptune populations would not simply be a matter of determining their size. Other aspects such as mass, temperature, and atmospheric properties also need to be examined before a candidate can be confidently designated as Hycean. But most of all, astronomers will need to get a good look at these potential Hyceans to see if there are any indications of biosignatures.
“It’s exciting that habitable conditions could exist on planets so different from Earth,” said Anjali Piette. Also exciting is the fact that the potential Hycean biosignatures that Dr. Madhusudhan and his team identified will be easier to detect using spectroscopic observations. Their larger sizes, higher temperatures, and hydrogen-rich atmospheres mean that any atmospheric signatures will be much more detectable than they would be with Earth-like planets. Said Madhusudhan:
“A biosignature detection would transform our understanding of life in the universe. We need to be open about where we expect to find life and what form that life could take, as nature continues to surprise us in often unimaginable ways.”
Other possible biosignatures include organic compounds like methyl chloride and dimethyl sulfide, which are less abundant on Earth but could be indicators of life on planets with hydrogen-rich atmospheres and low amounts of oxygen or ozone. Again, this is consistent with the “low hanging fruit” approach, where we search for biomarkers required for or produced by life as we know it.
When next-generation telescopes become available in the near future, the large sample of potential Hycean worlds prepared by the Cambridge team will be prime opportunities for follow-up observations. In addition, these planets are all relatively close by cosmic standards, orbiting M-type (red dwarf) stars located 35 to 150 light-years from Solar System. Already, there are plans to study K2-18b (the most promising candidate) using the next-generation James Webb Space Telescope (JWST).
When the JWST launches in November (or early Dec.) of this year, astronomers will be able to conduct Direct Imaging studies of exoplanets in the near- to mid-infrared wavelengths and obtain spectra directly from their atmospheres. The Nancy Grace Roman Space Telescope (RST) will follow in 2025, which will also conduct direct imaging studies using its advanced system of optics, coronographs, and spectrometers.
These results help to illustrate how exoplanet research has been growing and transitioning in recent years. With thousands of confirmed exoplanets now available for study, the process has moved from discovery to characterization. With improved instruments and methods, astronomers can place tighter constraints on what the planetary environments will be like.
In the midst of all this, scientists can test their theories about the kinds of conditions under which life could exist in our cosmos. After all, the ultimate goal is not to find just “life as we know it” but as it exists in all of its diversity and glory. Though we may be confined to looking for the low-hanging fruit right now, a day may come when we might be able to climb the tree of life and find what kind of exotic fruit grows farthest from the earth.
Further Reading: University of Cambridge, The Astrophysical Journal