We found our first exoplanets orbiting a pulsar in 1992. Since then, we’ve discovered many thousands more. Those were the first steps in identifying other worlds that could harbour life.
Now planetary scientists want to take the next step: studying exoplanet atmospheres.
The ESA’s ARIEL mission will be a powerful tool.
ARIEL stands for Atmospheric Remote-sensing Infrared Exoplanet Large-survey. It’s part of the European Space Agency’s Cosmic Vision Program. ARIEL’s goal is to examine the atmospheres of about 1,000 previously-confirmed exoplanets. It’ll study the chemical composition and the thermal structures of the atmospheres.
ARIEL is still in the design study phase, and its tentative launch date isn’t until 2028. Mission planners are still working out some of the mission’s critical details. One of those details includes automatic scheduling, and a new paper looks at those techniques and how the mission might work.
The paper is “Ariel mission planning: Scheduling the survey of a thousand exoplanets.” The lead author is Juan Carlos Morales, a researcher at the Institut de Ci`encies de l’Espai in Barcelona, Spain. The paper is available at the pre-press site arxiv.org.
NASA designed missions like Kepler and TESS to finding exoplanets, which have succeeded. But ARIEL’s mission is devoted to studying exoplanets. It’ll spend its time looking at known exoplanets rather than scanning the sky for more.
ARIEL will address several questions in exoplanet science. It’ll explore exoplanet composition, the formation and evolution of planetary systems, and the physical processes that shape exoplanet atmospheres.

Detailed knowledge of exoplanet atmospheres tells scientists how and where they formed. Planets form in protoplanetary disks, the disks of dust and gas that surround young stars. When scientists know about the chemical composition of an atmosphere and its thermal structure, they better understand where in the disk a planet formed and how quickly.
Science results from ARIEL can address other questions, too. One of those questions is how life got started on Earth.
Earth formed the same way exoplanets do: from a protoplanetary disk. But the evidence for life’s beginnings is long gone from Earth’s geological record. Observing terrestrial exoplanets can help answer the question of life’s origins. If ARIEL can show us how physical and chemical environments on planets similar to Earth evolved, we can get a de facto glimpse of early Earth, when life began.
But to accomplish any of this, ARIEL has to use its time wisely. That’s what this new paper explores.
“Automatic scheduling techniques are becoming a crucial tool for the efficient planning of large astronomical surveys,” the paper says.
Previous missions like Kepler and TESS were surveys. They surveyed a predetermined area of the sky, looking for transit signals. But ARIEL is different. It won’t watch a predetermined area of the sky but a predetermined list of existing targets. Since mission designers already know where those targets are, when they’ll transit in front of their stars, and when they’ll occult behind them, the mission can be scheduled more precisely and efficiently. But it also puts some stringent constraints on the planning since the mission isn’t based on passive observations.

The ESA is designing the ARIEL mission to last four years. “We conclude that Ariel will be able to fulfill the scientific objectives, i.e. characterizing ~1000 exoplanet atmospheres, with a total exposure time representing about 75–80% of the mission lifetime,” the authors write.
ARIEL will also watch some exoplanets during their complete orbits. It’ll watch their spectra vary as the planets orbit their stars. These are called phase curves, and they can give a more detailed picture of an exoplanet’s atmosphere than transits and occultations can.
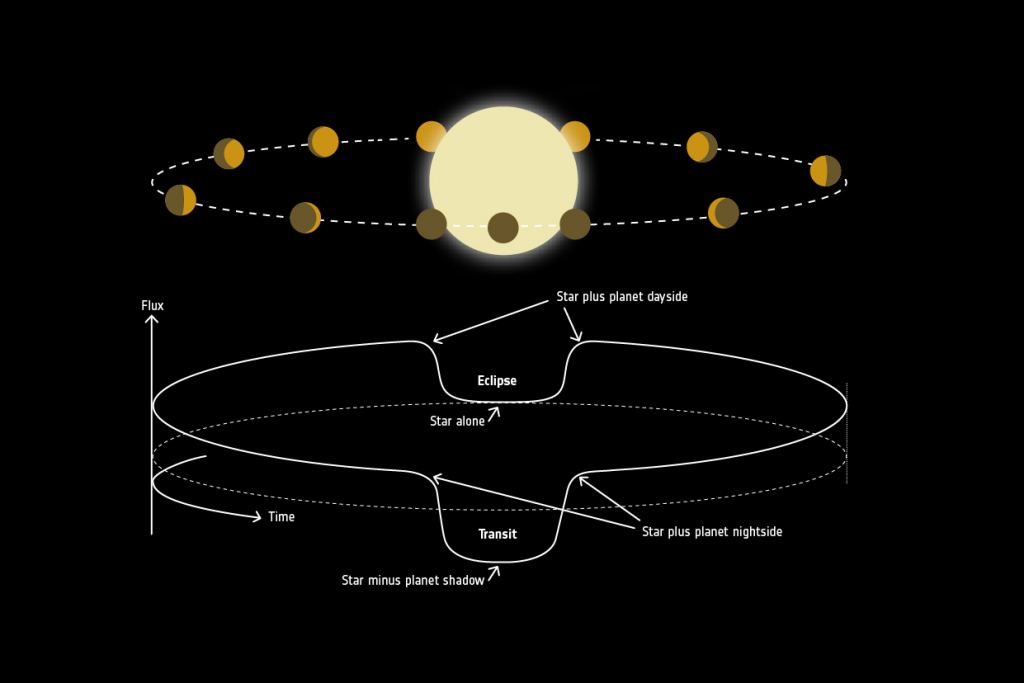
But ARIEL must balance more than transits, occultations, and phase curves. Other operations like maintaining the spacecraft’s orbit and auxiliary observations are also part of the scheduling equation. ARIEL’s instruments need periodic recalibration by observing bright G-type stars. Calibration could take up to 300 hours per year, about 3% of the mission. Station-keeping operations will take about four hours per month or 50 hours per year. That adds up to approximately 0.6% of the mission.
All of these factors put limitations on scheduling. In their paper, the authors discuss heuristics, algorithms, and related topics beyond the scope of this article. Their scheduling method involves a combination of Evolutionary Computation, Genetic Algorithms, Evolutionary Algorithms, and a subset of EC called SWARM intelligence. Interested readers can dig into the paper in more detail.
The guts of ARIEL’s schedule is the list of targets. In this new paper, the authors work with a Mission Reference Schedule (MRS), which is a combination of known exoplanet targets and targets yet to be found by TESS. They divided the targets into tiers based on the type of observations they want to do with the targets and how much observing time the targets require. For example, tier one targets include all of the planets in the MRS at low resolution spectra, and tier two is a ~600 planet subset of the whole MRS at medium resolution spectra. Tier three is 50 of the most interesting exoplanets orbiting bright stars that will be observed longer to get maximum resolution spectra.
Tier 3 targets have a higher priority than tier 2 targets, which have a higher priority than tier 1 targets. It’s easy to see how complicated the scheduling can get, and why the mission designers use AI and related methods to guarantee the most science results. But it’s even more complicated, because the mission designers hope they can complete other observations outside of the MRS.

Tier 4 planets are outside of the MRS, but are extremely desirable scientific targets. They’re short period systems and scientists want ARIEL to observe their phase curves. They’ll be scheduled in if it won’t affect the mission’s core science objectives. The authors have identified 43 targets in tier 4 that are further subdivided into three levels of priority.
Then there are back-up targets in case ARIEL can’t observe some of the planets in the MRS for various reasons. There are 1093 back-up exoplanets.
This paper gives an interesting behind the scenes look into mission scheduling, something most of us likely don’t spend much time thinking about. But precise and effective scheduling is critical to a mission’s success. The team’s simulations show that the ARIEL mission can be scheduled effectively enough to meet almost all of its science goals, and may even exceed them. Like every mission, there can be problems, delays, and unexpected difficulties.
“The main conclusion of the different simulations of the Ariel mission planning is that almost all the targets in the core sample can be observed as requested while meeting all mission and system requirements and constraints,” the team writes. “Only targets that are observable few times are challenging, and the scheduler can be used to easily identify them.”
The team points out that some inactive time slots are inevitable due to the complexity of the scheduling, and the hard and soft mission constraints. But even then, those time slots can still be maximized by re-observing some targets to get more detailed data. “In fact, by choosing wisely the targets to re-observe, it is possible to increase the number of well-characterized targets in the tier 3 subsample by a factor of ?2.5, or surveying several more Neptune and Earth-like planets considered as back-up targets.”

ARIEL will take exoplanet science a step further than where it is now. In a 2021 interview with Innovation News Network, ARIEL project scientist Theresa Lueftinger talked about some of the expectations for the ARIEL mission and exoplanet science overall. “I am sure we will find things we had not expected or even imagined before,” Lueftinger said. “That happens in science regularly, especially in exoplanet science. This is also what makes our life, our profession, and this field of science so exciting.”
ARIEL is just one of three ESA exoplanet missions. CHEOPS (CHaracterising ExOPlanet Satellite) launched in 2019 and is focusing on known exoplanets orbiting nearby bright stars. It’s observing planets in the super-Earth to Neptune size range. Its goal is to make high-precision observations of these planets to determine their bulk density.
PLATO (PLAnetary Transits and Oscillations of stars) will launch in 2026, and will search up to one million stars for exoplanet transits. PLATO’s goal is to discover rocky exoplanets around stars like our Sun, and sub-giant and red dwarf stars. The emphasis is on Earth-like planets around their stars’ habitable zone, where liquid water might exist on their surfaces.
More:
- Research Paper: Ariel mission planning: Scheduling the survey of a thousand exoplanets
- Research Paper: Ariel – a window to the origin of life on early earth?
- Universe Today: Cheops Finds a World That’s Utterly Alien From Anything We Have in the Solar System