Future historians might look back on this time and call it the ‘exoplanet age.’ We’ve found over 5,000 exoplanets, and we’ll keep finding more. Next, we’ll move beyond just finding them, and we’ll turn our efforts to finding biosignatures, the special chemical fingerprints that living processes imprint on exoplanet atmospheres.
But there’s more to biosignatures than atmospheric chemistry. On a planet with lots of plant life, light can be a biosignature, too.
The search for biosignatures on exoplanets got a boost of energy when the James Webb Space Telescope began observations. One of the telescope’s science objectives is to characterize exoplanet atmospheres with its powerful infrared spectrometry. If Webb finds large amounts of oxygen, for example, it’s an indication that biological processes might be at work and are changing a planet’s atmosphere. But the JWST and other telescopes could detect another type of biosignature.
Earth’s abundant plant life changes our planet’s ‘light signature.’ The change is based on photosynthesis and how plant life absorbs some light frequencies while reflecting others. The resulting phenomenon is called the vegetation red edge (VRE.)
Exoplanet scientists have worked on the idea of the VRE as a biosignature for a few years. It’s based on the fact that chlorophyll absorbs light in the visible part of the spectrum and is almost transparent in the infrared. Other cellular structures in the vegetation reflect the infrared. This helps plants avoid overheating during photosynthesis. This absorption and reflection make it possible for remote sensing to gauge plant health, coverage, and activity, and agricultural scientists use it to monitor crops.
In a new paper, a team of researchers looked at chlorophyll and its solar-induced fluorescence (SIF.) SIF is the name of the electromagnetic signal emitted by chlorophyll a, the most widely-distributed chlorophyll molecule. Part of the energy absorbed by chlorophyll a is not used for photosynthesis but is emitted at longer wavelengths as a two-peak spectrum. It covers roughly the 650–850 nm spectral range.

The paper is “Photosynthetic Fluorescence from Earth-Like Planets around Sun-Like and Cool Stars,” and it will be published in The Astrophysical Journal. The lead author is Yu Komatsu, a researcher at the National Institutes of Natural Sciences Astrobiology Center, National Astronomical Observatory of Japan.
The paper focuses on how the fluorescence from chlorophyll could be detected on planets similar to Earth. “This study examined the detectability of biological fluorescence from two types of photosynthetic pigments, chlorophylls (Chls) and bacteriochlorophylls (BChls), on Earth-like planets with oxygen-rich/poor and anoxic atmospheres around the Sun and M dwarfs,” the authors explain.
Detecting the presence of chlorophyll on another world is complicated. There’s a complex interplay between plant life, starlight, land/ocean coverage, and atmospheric composition. This study is part of an ongoing effort to understand some of the limitations to detection and what spectroscopic data can tell scientists about exoplanets. Over time, exoplanet scientists want to determine which detections can be biosignatures in different circumstances.

The VRE is a sharp drop in observed light between infrared and visible light. Light in the near-infrared (starting at about 800 nm) is much brighter than the light in the optical (between about 350 to 750 nm.) On Earth, this is the light signature of plant life and its chlorophyll. The chlorophyll absorbs the light up to 750 nm, and other plant tissues reflect light above 750 nm.
Satellites like NASA’s Terra can observe different regions on Earth’s surface over time and watch how the light reflectance changes. Scientists measure what’s called the Normalized Difference Vegetation Index (NVDI.) A dense forest location during peak growing season gives peak values for the NDVI, while vegetation-poor regions give low values.
Scientists can also observe Earthshine, the light reflected from Earth onto the Moon. That light is the entirety of the light reflected by Earth, what scientists call a disk-averaged spectrum. “Whereas remote sensing observes local areas on Earth, Earthshine observations provide disk-averaged spectra of the Earth, leading to fruitful insights into exoplanet applications,” the authors write. “The apparent reflectance change in the Earth’s disk-averaged spectrum due to surface vegetation is less than 2%.”
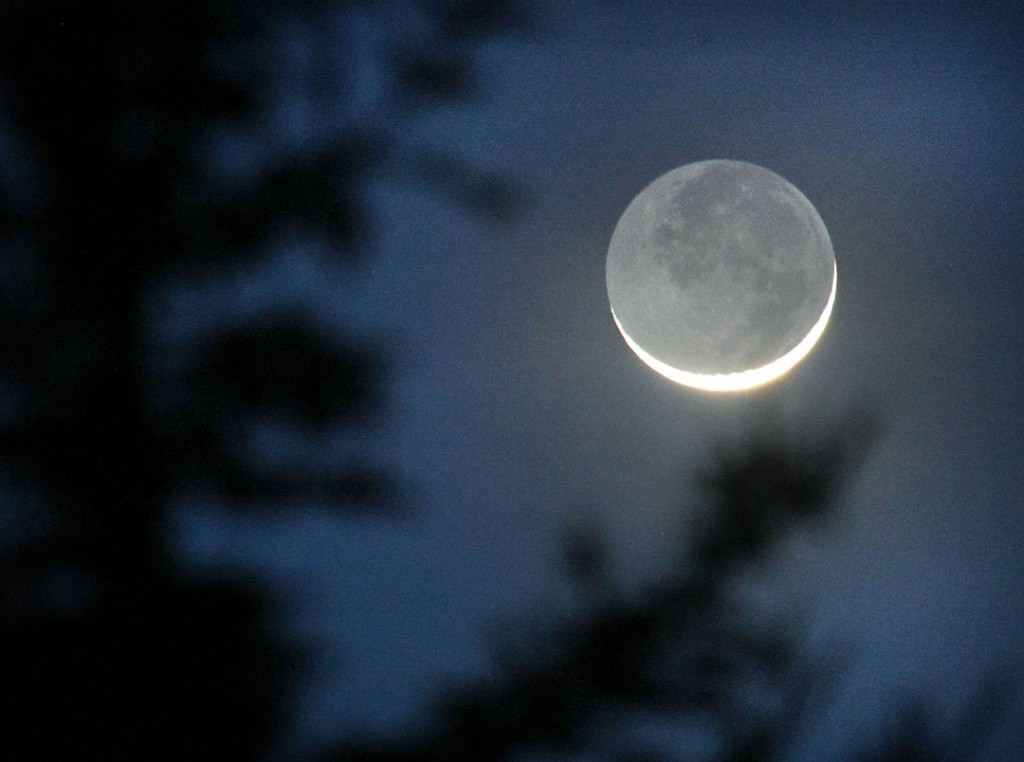
The Earthshine we see on the Moon is similar to the light we detect from distant exoplanets. It’s the totality of the light vs regional surface light. But there’s an enormous amount of complexity involved in studying that light, and there are no easy comparisons between Earth and exoplanets. “The VRE signals from exoplanets around stars other than a Sun-like star are challenging to predict due to the complexity of photosynthetic mechanisms in different light environments,” the authors explain. But there’s still value in looking for a VRE on exoplanets. If scientists observe an exoplanet frequently, they may be able to recognize how the VRE changes seasonally, and they may recognize a similar VRE-like step in the planet’s spectroscopy, though it could be at different wavelengths than on Earth.
In their paper, the researchers considered an Earth-like planet in different stages of atmospheric evolution. In each case, the planets orbited the Sun, a well-studied red dwarf named Gliese 667 C, or the even more well-known red dwarf TRAPPIST-1. (Both red dwarfs have planets in their habitable zones, and both represent common types of red dwarfs.) They modelled the reflectance from each case for vegetation chlorophyll, bacterial chlorophyll-based vegetation, and biological fluorescence without any surface vegetation.
What they came up with is a collection of light curves that shows what different VREs might look like on Earth-like exoplanets in different stages of atmospheric evolution around different stars. It’s important to look at different stages of atmospheric evolution because Earth’s atmosphere changed from oxygen-poor to oxygen-rich while life was present.
“We considered fluorescence emissions from Chl- and BChl-based vegetation in a clear-sky condition
on an Earth-like planet around the Sun and two M dwarfs,” the authors write.

The study produced a range of reflectance data for Earth-like planets around different stars. The planets were modelled with different atmospheres that correspond to Earth’s different atmospheres over its four billion-year history. The researchers also varied the amount of land cover vs ocean cover, the amount of coastline, and whether the surface was covered in plants or in photosynthetic bacteria.
In the future, we’ll be wielding ever more powerful space telescopes like LUVOIR (Large UV/Optical/IR Surveyor) and HabEx (Habitable Exoplanet Observatory.) Ground-based telescopes like the Thirty Meter Telescope, the Giant Magellan Telescope, and the European Extremely Large Telescope will also be coming online in the near future. These telescopes are going to generate an unprecedented amount of data on exoplanets, and this study is part of preparing for that.

We’re detecting more and more exoplanets and are building a statistical understanding of other solar systems and the distributions, masses, and orbits of exoplanets. The next is to gain a deeper understanding of the characteristics of exoplanets. Telescopes like the E-ELT will do that with its 39.3-meter mirror. It’ll be able to separate an exoplanet’s light from the star’s light and directly image some exoplanets. It’ll unleash a flood of data on exoplanet reflectance and potential biosignatures, and all of that data will have to be evaluated.
If we ever locate an Earth-like planet, one that’s habitable and currently supporting life, it won’t just appear in one of our telescopes and announce its presence. Instead, there’ll be tantalizing hints, there’ll be indications and contra-indications. Scientists will slowly and carefully work their way forward, and one day we might be able to say we’ve found a planet with life. This research has a role to play in the endeavour.
“It is important to quantitatively evaluate the detectability of any potential surface biosignature using expected specifications of specific future missions,” the authors explain. “This study made the first attempt to investigate the detectability of photosynthetic fluorescence on Earth-like exoplanets.”
More:
- New Research: Photosynthetic Fluorescence from Earth-Like Planets around Sun-Like and Cool Stars
- Older Research: Vegetation’s red edge: a possible spectroscopic biosignature of extraterrestrial plants
- Universe Today: Habitable Planets Around Red Dwarf Stars Might not get Enough Photons to Support Plant Life