In 1992, humanity’s effort to understand the Universe took a significant step forward. That’s when astronomers discovered the first exoplanets. They’re named Poltergeist (Noisy Ghost) and Phobetor (Frightener), and they orbit a pulsar about 2300 light-years away.
Even though we thought there must be other planets around other stars, and entire science fiction franchises were built on the idea, we didn’t know for sure and couldn’t just assume it to be true. A quick glance at human history shows how wrong our assumptions about nature can be.
Since then, thanks largely to NASA’s Kepler and TESS missions, a flood of exoplanet discoveries has confirmed our assumptions about planets in other solar systems. But while we assumed that other solar systems would be much like ours—we had nothing else to go by—the 5000+ exoplanets we’ve discovered have shown us the folly of our assumptions.
We can’t be blamed for the assumption that other solar systems would be similar to ours. It makes sense that rocky planets would be closest to the star, and gas giants and ice giants would be further away. Even the nice tidy boundary provided by the main asteroid belt makes sense. It also makes sense that planets would orbit their star on the ecliptic with a little variation, just like the planets in our system.
But instead, astronomers have found a preponderance of gas giants, including the hot Jupiters. In fact, the first exoplanet discovered around a Sun-like star was a hot Jupiter that orbited its star in only four days. A lot of that can be chalked up to detection bias in the transit method, which accounts for the majority of planet detections.

Our assumptions about orderly solar systems similar to ours are well in our rear-view mirror now, as we’ve discovered exoplanets on wildly eccentric orbits, exoplanets in places we never expected them, like in orbit around white dwarfs, and planets so bizarre that molten iron rain might fall from the sky.
But there’s a sub-class of exoplanets that’s garnering more attention from exoplanet scientists. These planets are in polar orbits around their stars. A team of astronomers has found another one, and the discovery is begging for an explanation.
Astronomers use the Rossiter-McLaughlin effect to determine which way a star is rotating and if an exoplanet is in a polar orbit. It’s based on redshift and blueshift. The side of a star rotating towards us is approaching us, and the light from that part of the Sun will shift into blue. The side rotating away from us shifts its light into the red. As a planet transits in front of the star, it affects the shift, and astronomers can measure the effect.

The researchers presented their work in a new paper that will be published in the journal Astronomy and Astrophysics. It’s titled “A puffy polar planet: The low-density, hot Jupiter TOI-640 b is on a polar orbit.” The lead author is Emil Knudstrup, a Ph.D. student in the Department of Physics and Astronomy at Aarhus University, Denmark. Another of the authors, Simon Albrecht, is known for researching exoplanets in polar orbits and is an author and co-author of other papers on the topic.
TOI-640 is a main-sequence F-type star. It’s about 1.5 times more massive than the Sun and about double the radius. The star is about 2 billion years old and is about 1115 light-years away from us. TOI-640 is a binary star, and its companion is a red dwarf.

TOI-640 b is a hot, puffy Jupiter. It has about 60% of the mass of Jupiter and a radius of about 1.7 of Jupiter’s. But what makes the planet stand out is its stellar obliquity. Stellar obliquity is the difference between a star’s spin axis and the orbit of its planets. TOI-640 has a stellar obliquity of 184 ± 3?. That means that the planet TOI-640 b is in a polar orbit around the star.
And TOI-640 b isn’t the only one.
There are far too many planets like this to just ignore them as irregularities. Research shows while most hot Jupiters follow orbits aligned with their star, a significant number have misaligned orbits. Those with misaligned orbits tend to have polar orbits.
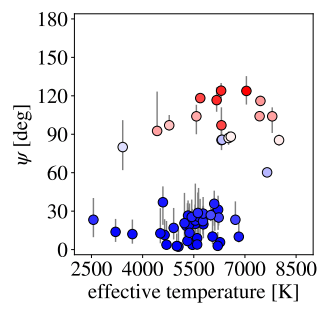
It’s interesting that misaligned orbits don’t span the range of obliquities. Instead, they tend to clump up in polar orbits, which can’t be a fluke. In a 2021 paper titled “A Preponderance of Perpendicular Planets,” the authors wrote that the “pile-up of polar orbits is a clue about the unknown processes of obliquity excitation and evolution.”
In the same 2021 paper, researchers outlined four possible causes for planets in polar orbits and why there’s a tendency for misaligned planets to enter polar orbits.
Tidal Dissipation: Astronomers think that TD will usually dampen the obliquity, but in some cases, it can cause the obliquity to linger at 90?. That happens when the damping is dominated by the dissipation of inertial waves driven in the convective zone by Coriolis forces. But some stars with planets in polar orbits lack convective zones, and others have such wide separations between them and their planets that the effect of TD is negligible.
Kozai Mechanism: These are interactions between a star and its planet and a third body called the perturber. It can affect inclination and eccentricity and can even flip planets into retrograde or prograde orbits. TOI-640 has a red dwarf partner star which could act as a perturber.
Secular Resonance Crossing: This takes place early in the solar system’s history when the disk is still prominent. The resonance between the transiting planet and an outer companion decreases the disk mass. It excites the inclination of the inner planet and pushes it to 90?.
Magnetic Warping: This can tilt the whole protoplanetary disk toward a perpendicular orientation. But other things can counteract it, like magnetic braking and disk winds.

The authors point out that these mechanisms can explain some of the polar orbits they see but not all of them. “While these mechanisms might be able to explain parts of the observed distribution, they do not seem to be able to fully reproduce the observations individually,” they write.
But all of these mechanisms can account for planets on polar orbits. Nature doesn’t need to rely on only one of them. “It would be interesting to increase the sample size and expand the parameter space to try to decipher whether or not these mechanisms work in tandem in different types of systems harbouring different types of planets,” they write.
As astronomers learn more about other solar systems, the details of which mechanisms dominate at what times and under what conditions will become clearer. Maybe their discoveries will test more of our assumptions about other solar systems.
More:
- Paper: A puffy polar planet: The low-density, hot Jupiter TOI-640 b is in a polar orbit.
- Research Article: A Preponderance of Perpendicular Planets
- The Conversation: Stars with planets on strange orbits: what’s going on?
- Universe Today: This Hellish Planet Orbits its Star Every 18 Hours. How Did it Get There?