The Kuiper Belt, the vast region at the edge of our Solar System populated by countless icy objects, is a treasure trove of scientific discoveries. The detection and characterization of Kuiper Belt Objects (KBOs), sometimes referred to as Trans-Neptunian Objects (TNOs), has led to a new understanding of the history of the Solar System. The disposition of KBOs is an indicator of gravitational currents that have shaped the Solar System and reveal a dynamic history of planetary migrations. Since the late 20th century, scientists have been eager to get a closer look at KBOs to learn more about their orbits and composition.
Studying bodies in the outer Solar System is one of the many objectives of the James Webb Space Telescope (JWST). Using data obtained by Webb’s Near-Infrared Spectrometer (NIRSpec), an international team of astronomers observed three dwarf planets in the Kuiper Belt: Sedna, Gonggong, and Quaoar. These observations revealed several interesting things about their respective orbits and composition, including light hydrocarbons and complex organic molecules believed to be the product of methane irradiation.
The research was led by Joshua Emery, a Professor of Astronomy and Planetary Sciences at Northern Arizona University. He was joined by researchers from NASA’s Goddard Space Flight Center (GSFC), the Institut d’Astrophysique Spatiale (Université Paris-Saclay), the Pinhead Institute, the Florida Space Institute (University of Central Florida), the Lowell Observatory, the Southwest Research Institute (SwRI), the Space Telescope Science Institute (STScI), American University. and Cornell University. A preprint of their paper has appeared online and is being reviewed for publication by Icarus.
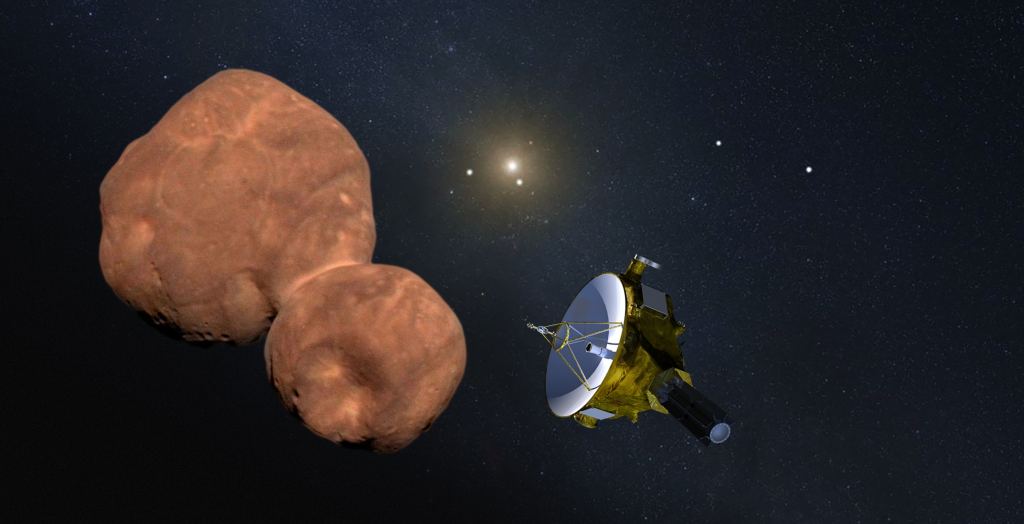
Despite all of the advances in astronomy and robotic explorers, what we know about the Trans-Neptunian Region and the Kuiper Belt is still limited. To date, the only mission to study Uranus, Neptune, and their major satellites was the Voyager 2 mission, which flew past these ice giants in 1986 and 1989, respectively. Moreover, the New Horizons mission was the first spacecraft to study Pluto and its satellites (in July 2015) and the only one to encounter an object in the Kuiper Belt, which occurred on January 1st, 2019, when it flew past the KBO known as Arrokoth.
This is one of the many reasons why astronomers have eagerly awaited the launch of the JWST. In addition to studying exoplanets and the earliest galaxies in the Universe, its powerful infrared imaging capabilities have also been turned toward our backyard, revealing new images of Mars, Jupiter, and its largest satellites. For their study, Emery and his colleagues consulted near-infrared data obtained by Webb of three planetoids in the Kuiper Belt – Sedna, Gonggong, and Quaoar. These bodies are about 1,000 km (620 mi) in diameter, which places them within the IAU designation for Dwarf Planets.
As Emery told Universe Today via email, these bodies are especially interesting to astronomers because of their size, orbits, and compositions. Other Trans-Neptunian bodies – like Pluto, Eris, Haumea, and Makemake – have all retained volatile ices on their surfaces (nitrogen, methane, etc.). The one exception is Haumea, which lost its volatiles in a large impact (apparently). As Emery said, they wanted to see if Sedna, Gonggong, and Quaoar have similar volatiles on their surfaces as well:
“Previous work has shown that they may be able to. While all being roughly similar sizes, their orbits are distinct. Sedna is an inner Oort Cloud object with perihelion of 76 AU and aphelion of nearly 1,000 AU, Gonggong is in a very elliptical orbit also, with perihelion of 33 AU and aphelion ~100 AU, and Quaoar is in a relatively circular orbit near 43 AU. These orbits place the bodies in different temperature regimes and different irradiation environments (Sedna, for instance, spends most of its time outside the Sun’s heliosphere). We wanted to investigate how those different orbits could affect the surfaces. There are also other interesting ices and complex organics on the surfaces.”

Using data from Webb’s NIRSpec instrument, the team observed all three bodies in low-resolution prism mode at wavelengths spanning 0.7 to 5.2 micrometers (µm) – placing them all in the near-infrared spectrum. Additional observations were made of Quaoar from 0.97 to 3.16 ?m using medium-resolution gratings at ten times the spectral resolution. The resulting spectra revealed some interesting things about these TNOs and the surface compositions, said Emery:
“We found abundant ethane (C2H6) on all three bodies, most prominently on Sedna. Sedna also shows acetylene (C2H2) and ethylene (C2H4). The abundances correlate with the orbit (most on Sedna, less on Gonggong, least on Quaoar), which is consistent with relative temperatures and irradiation environments. These molecules are direct irradiation products of methane (CH4). If ethane (or the others) had been on the surfaces for a long time, they would have been converted to even more complex molecules by irradiation. Since we still see them, we suspect that methane (CH4) must be resupplied to the surfaces fairly regularly.”
These findings are consistent with those presented in a pair of recent studies led by Dr. Will Grundy, an astronomer with the Lowell Observatory and a co-investigator on NASA’s New Horizons mission, and Chris Glein, a planetary scientist and geochemist at the SwRI. For both studies, Grundy, Glien, and their colleagues measured deuterium/hydrogen (D/H) ratios in methane on Eris and Makemake and concluded that the methane was not primordial. Instead, they argue that the ratios result from methane being processed in their interiors and delivered to the surface.
“We suggest the same may be true for Sedna, Gonggong, and Quaoar,” said Emery. “We also see that the spectra of Sedna, Gonggong, and Quaoar are distinct from those of smaller KBOs. There were talks at two recent conferences that showed JWST data of smaller KBOs cluster into three groups, none of which look like Sedna, Gonggong, and Quaoar. That result is consistent with our three larger bodies having a different geothermal history.”
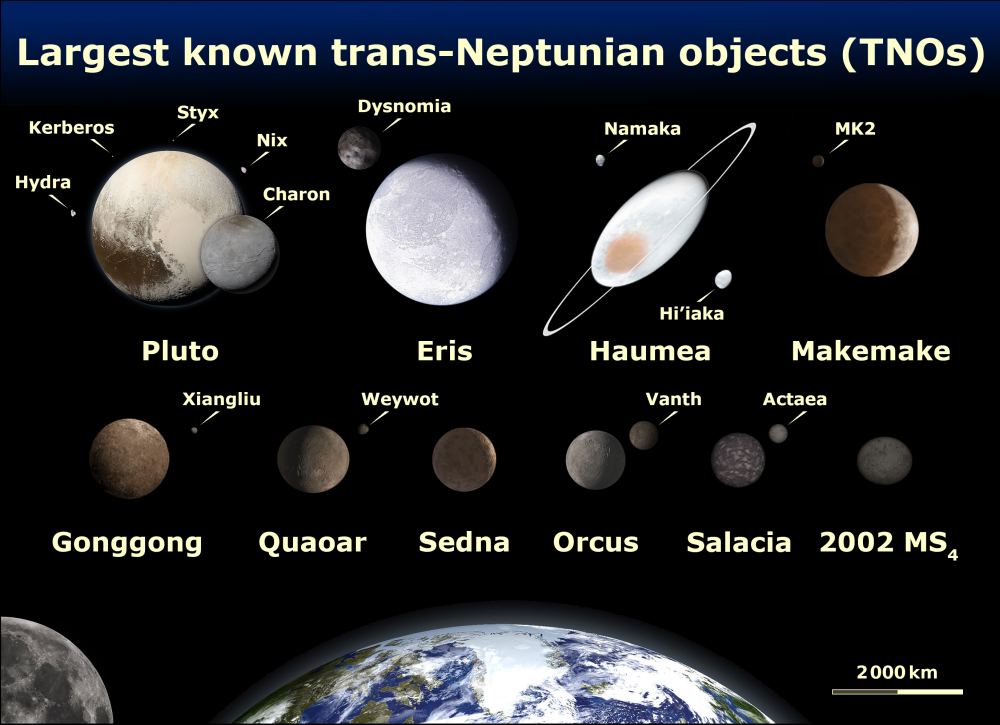
These findings could have significant implications for the study of KBOs, TNOs, and other objects in the outer Solar System. This includes new insight into the formation of objects beyond the Frost Line in planetary systems, which refers to the line beyond which volatile compounds will freeze solid. In our Solar System, the Trans-Neptunian region corresponds to the nitrogen line, where bodies will retain large amounts of volatiles with very low freezing points (i.e., nitrogen, methane, and ammonia). These findings, said Emery, also demonstrate what type of evolutionary processes are at work for bodies in this region:
“The primary implication may be finding the size at which KBOs have become warm enough for interior reprocessing of primordial ices, perhaps even differentiation. We should also be able to use these spectra to better understand irradiation processing of surface ices in the outer Solar System. And future studies will also be able to look in more detail at volatile stability and the possibility for atmospheres on these bodies over any parts of their orbits.”
This study’s results also showcase the abilities of the JWST, which has proven its worth many times since it became operational early last year. They also remind us that in addition to enabling new visions and breakthroughs of distant planets, galaxies, and the large-scale structure of the Universe, Webb can also reveal things about our little corner of the cosmos.
“The JWST data are fantastic,” added Emery. “They enabled us to get spectra at longer wavelengths than we can from the ground, which enabled the detection of these ices. Often, when observing in a new wavelength range, the initial data can be pretty poor quality. JWST not only opened up a new wavelength range but also provided fantastically high-quality data that are sensitive to a suite of materials on the surfaces in the outer Solar System.”
Further Reading: arXiv