Despite the fact that we’ve discovered thousands of them, exoplanets are hard to find. And some types are harder to find than others. Naturally, some of the hardest ones to find are the ones we most want to find. What can we do?
Keep working on it, and that’s what a trio of Chinese scientists are doing.
Scientists have four methods of detecting exoplanets. The transit method is the most prolific, but there’s also the radial velocity method, astrometry, and, of course, direct imaging. Each one works differently, has different strengths and weaknesses, and is better at finding some planets around different types of stars than others.
We’re mostly interested in finding terrestrial planets in the habitable zones of nearby stars. Which of the four methods will deliver the best results as we continue our search?
The three Chinese scientists set out to determine that. Their paper “The Potential of Detecting Nearby Terrestrial Planets in the HZ with Different Methods,” sums up their results. It appeared in the Publications of the Astronomical Society of the Pacific. The lead author is Hao Qiao-Yang from the School of Astronomy and Space Science, Nanjing University, China.
“Terrestrial planets in the habitable zone around nearby stars are of great interest and provide a good sample for further characteristics of their habitability,” the authors write. The more of them we can find, the better we can understand them, and then we can use that understanding to find even more of them. But this research didn’t involve any actual planet hunting.

The researchers dipped into Gaia’s Catalog of Nearby Stars to obtain a sample of 2,234 main sequence stars within 20 parsecs, or about 65 light years. The sample excluded brown dwarfs and white dwarfs. Then they derived the extended habitable zones (HZ) for all those stars. Finally, they performed simulations by injecting Earth-like planets into each HZ.
That set the stage for the final piece. They determined what signals each of these 2,234 planets would send that would be recieved by each of the four exoplanet detection methods: velocity amplitude for radial velocity, transit probability and depth for the transit method, stellar displacements for astrometry, and contrast and angular separation for imaging. Then, they “… predict the highest possible detection number of Earth-like planets via different methods in the best-case hypothetical scenario.”
Why the focus on nearby stars? Well, no matter what method planet-hunters use, exoplanets are easier to find the closer they are, and also easier to confirm. They’re also easier to study with follow-up observations by telescopes like the JWST, which can characterize exoplanet atmospheres and detect potential biosignatures.
Determing the habitable zones around all these stars was tricky. Impossible, in fact. “An HZ is rarely determined precisely because it depends on not only on the stellar spectrum and stellar activity, but also on the planetary atmosphere,” the authors write. To simplify things, they used extended habitable zones, which increases the occurrence rate of planets in the HZ. The inner boundary (IHZ) is set to “Recent Venus” and the outer boundary (OHZ) is set to “Early Mars.”
“Since we adopt the extended HZ, we assume an occurrence rate of Earth-like planets in the HZ of 1, and put one Earth-like planet in the HZ around every single star,” the authors explain. This doesn’t mean they expect there to be this many of these desirable exoplanets. It just gives them a more complete sample to work with, and that produces better results.
“The average location of IHZ and OHZ is 0.22 and 0.43 au, respectively,” the paper states. That may sound awfully close, but most stars are cool M dwarfs, and their HZs are closer than our Sun’s, which is a much more luminous main sequence star.
The meat of the study concerns the signals we’d receive from all of these planets with the four detection methods.
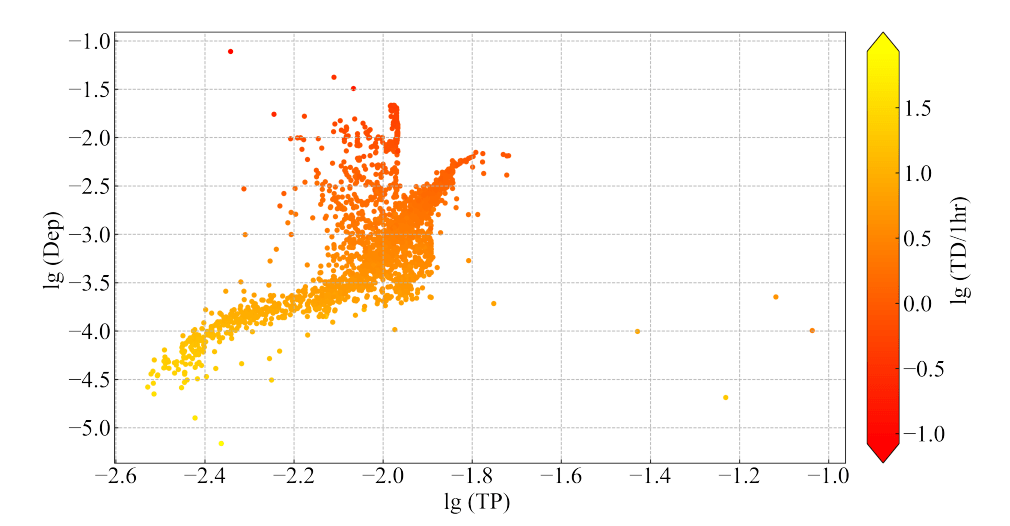
The transit method has been our most prolific exoplanet detection method, and the probability of detecting a signal reflects that. However, the transit method only works when the geometry is right. The transiting planet has to pass between its star and us. The other methods don’t have the same limitation, though the RV method isn’t totally immune from geometry.
Of all the planets detected in the simulations, the transit method first detected 24 of them. However, since the transit method excels at finding planets close to their stars, all 24 of them were too close to their stars and not inside the HZ.
The study’s results show that the radial velocity method is most effective. Our most sensitive RV instrument is the ESO’s ESPRESSO, or Echelle SPectrograph for Rocky Exoplanets and Stable Spectroscopic Observations. Since it’s our best instrument, the researchers used its sensitivity in their simulations. How easily could ESPRESSO detect the planets in the sample?
Of the 252 planets detected within the study’s 20 parsec radius, the RV method using ESPRESSO is responsible for 221 of them. Four of them are Earth-like.
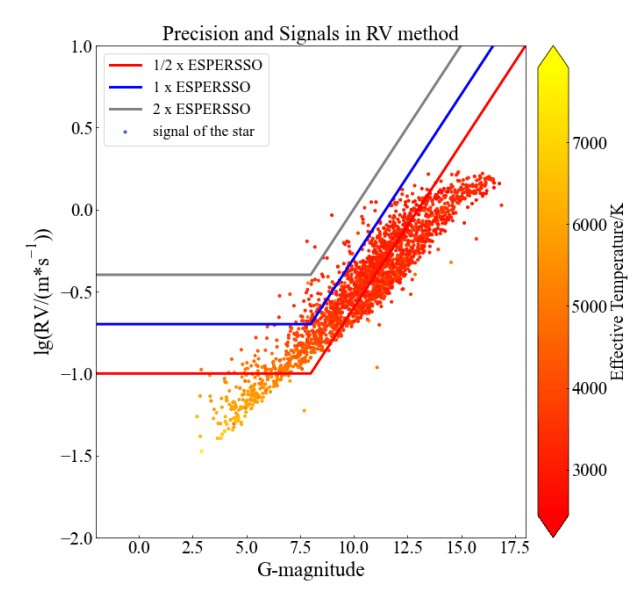
The astrometry method is one of the most sensitive ways to detect exoplanets. It relies on detecting the minute wobbles in host stars created by their orbiting planets. It’s how the ESA’s Gaia mission works, and in the near future, several more observatories will use the astrometry method. The simulations show that astrometry prefers finding planets in the HZ around massive stars, but that current astrometry is not sensitive enough to detect about 98% of planets within 20 parsecs.
There’s a critical distinction between Gaia’s astrometry method, and astrometry used to find exoplanets. Gaia measure stars using absolute astrometry, but finding exoplanets uses relative astrometry. Astrometry measures displacement, which is measured in microarcseconds. “We have found that more than 98% of the maximum stellar displacements of stars within 20 pc are under 1 ?as,” the authors write. That means we need greater precision to detect more exoplanets.
The figure below shows how more precise astrometry detects more planets.
The least precise gray line detects five habitable planets, the blue line is more precise and the expected number increases to 30, including seven planets around G stars and 10 planets around M stars. The red line represents the most precise astrometry and the expected number becomes 511, including 116 and 174 planets around G and M stars, respectively.
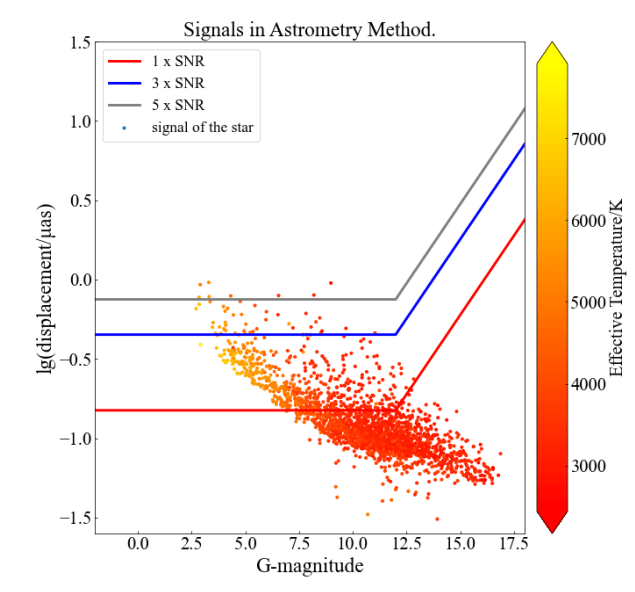
The astrometry method has the greatest potential to detect Earth-like planets in HZs around Sun-like stars, but only with better precision.
Lastly, the researchers simulated results from direct imaging, a method only in its infancy. It works best on young planets still emitting infrared that are far from their stars. There are sub-types of direct imaging, and together they’ve found about 30 planets.
Planet hunting scientists have found six exoplanets within 20 parsecs with direct imaging. That may not sound great, but the method has some advantages over RV and the transit method. It’s not sensitive to an exoplanet’s inclination. But compared to the other methods, direct imaging is more complex because it needs to take both contrast and angular resolution into account. Direct imaging can use both optical and infrared light.
The results show that direct imaging excels at detecting planets around G-type or Sun-like stars.
“In the optical band, the predicted number of exoplanets is 159, and 92 of them are around G stars,” the authors write. Infrared direct imaging is even more productive, finding 191 exoplanets, with 106 of them around G-type stars.
Method | Reference Instrument | Expected HZ Planets (known, expected, around G stars) |
Radial Velocity | ESPRESSO | 4, 39,1 |
Transit | TESS | 4, 5, 0 |
D.I. Optical | LUVOIR | 0, 159, 92 |
D.I. Infrared | LIFE | 0, 191, 96 |
Astrometry | 3xGaia | 0, 30, 8 |
When it comes to finding exoplanets in HZs around nearby stars, each method has its strengths and weaknesses and is better at finding planets around different types of stars.
The paper is a preliminary effort to compare the potential of the different detection methods. Out of necessity, it’s based on some ideal assumptions and in part on the improved sensitivity of future instruments. Its purpose is to help develop a framework for “… selecting the targets and designing the precision requirements of the four different methods.”
The hunt for exoplanets shows no signs of slowing down. Why would it? Finding Earth-like planets in habitable zones is one of our most meaningful scientific pursuits.
The simulation is based on terrestrial planets existing in the HZs of every main sequence star within 20 parsecs. So the specific numbers in the results are likely not accurate. But that isn’t really the point.
Future instruments will out of necessity be more sensitive, including LUVOIR and HabEx. So this study shows what could be possible, and lays the groundwork for how we can use future instruments with the greatest success.