Supernovae are relatively rare. It might not seem like it, but that’s because they’re so bright we can see them in other galaxies a great distance away. In fact, in 2022, astronomers spotted a supernova over 10 billion light-years away.
Any time astronomers spot a supernova, it’s an opportunity to learn more about these rare, cataclysmic explosions. It’s especially valuable if astronomers can get a good look at the progenitor star before it explodes.
We know what types of stars explode as core-collapse supernovae: massive ones. But we don’t know which star will explode when, so we don’t know where to look to see the progenitor. The authors of new research put this succinctly when they write, “Obtaining spectroscopic observations of the progenitors of core-collapse supernovae is often unfeasible due to an inherent lack of knowledge as to which stars will go supernova and when they will explode.”
That quote comes from a new research letter titled “Spectroscopic observations of progenitor activity 100 days before a Type Ibn supernova.” The letter has been submitted to the journal Astronomy and Astrophysics and is currently in pre-press. The lead author is Seán Brennan from the Department of Astronomy at Stockholm University.
Astronomers keep learning more about supernovae progenitors. They’re finding that supernova progenitors can exhibit powerful outbursts in the weeks, months, or even years before they explode. Astronomers keep getting better and better tools to spot these outbursts, and sometimes they get lucky.
In April of 2023, a massive star exploded in NGC 4388, a spiral galaxy about 57 million light-years away. The Zwicky Transient Facility (ZTF) spotted it, and the supernova is called SN 2023fyq. SN 2023fyq is a rare type of supernova called a Type Ibn. They show a lack of hydrogen lines and narrow He I emission lines in their spectra. Astronomers think that these characteristics come from the SN interacting with hydrogen-poor, helium-rich circumstellar material (CSM.)
Astronomers only know of a few of these types of SN, so their progenitors are poorly understood. Prior to its explosion, the ZTF also spotted the precursor activity, providing a window into these mysterious progenitors.
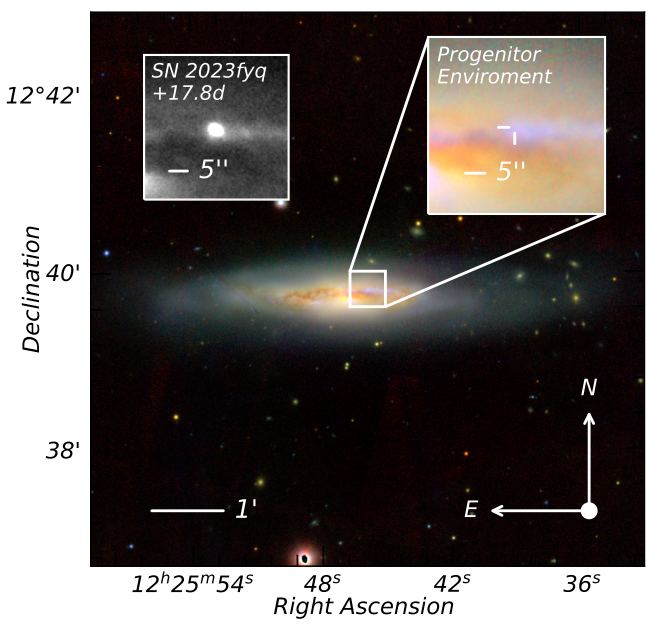
“This Letter presents spectral and photometric observations of the progenitor of a Type Ibn SN several months before core-collapse, as well as SN 2023fyq itself,” the researchers write. The observations come from multiple telescopes and observatories, including the Keck 10m telescope, the Palomar 200-inch telescope, and the Gemini North 8m telescope.
The researchers found that the progenitor’s luminosity increased exponentially during the 150 days leading up to the explosion. They also found that the radius of the photosphere remained almost constant during the same time. The pre-supernova spectra also “reveal a complex evolving He I profile.”
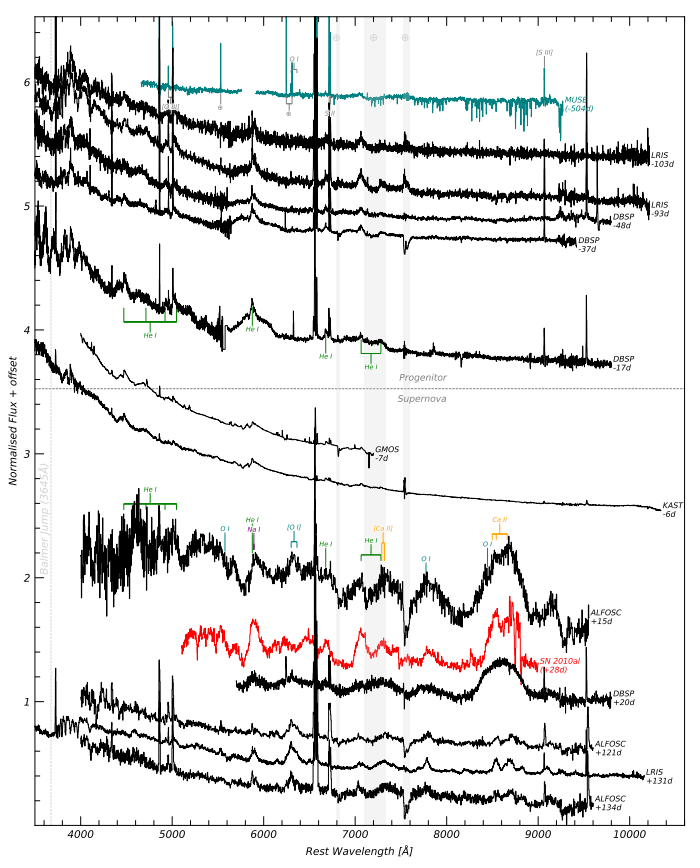
The He I profile could be a clue to some of the progenitor star’s activity. There are similar He I emissions in both the progenitor and the SN. “This would mean that the asymmetric material responsible for this emission was not destroyed in the SN explosion,” the authors explain. “SN ejecta interacting with asymmetric circumstellar material (CSM) has been used to explain irregular emission line profiles.” We’re getting deep into the weeds here, but it’s significant. “… SN 2023fyq provides the first clear spectroscopic evidence of asymmetric structure prior to core-collapse.”
It’s possible that some of the features in the spectroscopy are caused by circumstellar material (CSM). “Some mechanisms cause the progenitor to be surrounded by a dense CSM,” the authors explain, “and may lead to shock dissipation and emission of radiation in the optically thick CSM.” In that case, diffusion could explain the light curve’s general rise. “This also explains the roughly constant radius and the slowly rising effective temperature,” they write.
“These observations of SN 2023fyq and the final moments of the progenitor highlight that the progenitors to CCSNe can undergo some extreme instabilities shortly before their final demise,” the authors write.
It shouldn’t surprise anyone that a progenitor exhibited some extreme instabilities before exploding as a supernova. It would be very strange if a massive star suddenly exploded with no lead-up. Only massive stars explode as supernovae, and it happens when the star’s outward fusion pressure is insufficient to counteract the star’s own gravity. The star collapses in on itself and explodes. This is a cataclysmic event, and there are bound to be shock waves travelling through the star, as well as other interactions. There are bound to be “extreme instabilities,” as the authors call them.
But what exactly does this tell us?
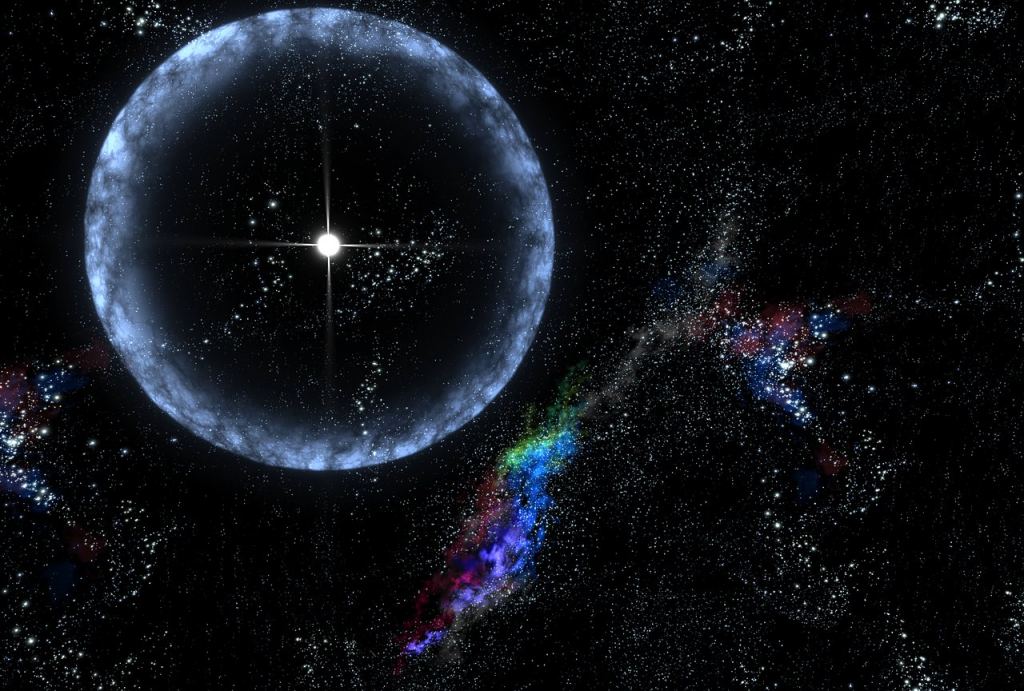
This is just a research letter, and the authors are presenting their results to the astronomical community. They can show the unusual activity evident in spectroscopic observations, but they can’t tell us exactly what it means yet. But it does show that we’re able to spot supernova progenitors, a huge step in understanding core-collapse supernovae.
“Progenitor analysis typically occurs after the star has been destroyed by searching through archival images and measuring the photometric properties of the assumed progenitor,” the researchers write in their letter. “Although this area of transient astronomy is in its infancy, the repercussions of detecting precursor activity are immense, highlighting that the progenitor is not in an equilibrium state and may
not be represented well by standard stellar evolutionary models.”
We’re looking at an SN progenitor when we look at Betelgeuse; astronomers just don’t know how long it’ll be until the star explodes. But it appears to have belched plasma that created a dust cloud that briefly dimmed the star a couple of years ago. Is that behaviour indicative of how other progenitors behave?
Astronomers need to observe more supernova progenitors of different types before they can answer their questions. Once they have more data, they’ll build models of how supernova progenitors behave leading up to the explosion. Then, they can observe even more SN and test that data against their models. Then, they’ll improve their models some more.
Eventually, they’ll have answers.
Typo alert:
The text states the SN occurred in NGC 4388, while the caption of the corresponding image states that the SN occurred in NGC 3288.