Gamma-ray telescopes observing neutron star collisions might be the key to identifying the composition of dark matter. One leading theory explaining dark matter it that is mostly made from hypothetical particles called axions. If an axion is created within the intensely energetic environment of two neutron stars merging, it should then decay into gamma-ray photons which we could see using space telescopes like Fermi-LAT.
About 130 million years ago, a pair of neutron stars collided violently. The powerful gravitational waves from the impact radiated outwards at the speed of light, followed shortly after by a tremendous flash of radiation. On 17 August 2017, the gravitational waves reached Earth, and were detected by both detectors of the Laser Interferometer Gravitational-Wave Observatory (LIGO) in the United States, and the Virgo interferometer in Italy. This event was named GW170817. Mere seconds later, the Fermi-LAT gamma ray telescope recorded a burst of gamma rays in the same region of sky. Over the next few days, other telescopes saw and recorded the event in visible light and other wavelengths. This marked the first ever multi-messenger observation of two neutron stars merging.
What is an axion?
One of the leading theories around the composition of dark matter is that it is mostly made from a hypothetical particle called an axion. If enough axions were created in the big bang, and if their masses fall within a specific range, then they could account for much of the dark matter shaping the universe today. Unfortunately, axions have never been observed, and nobody has yet confirmed whether they even exist. But according to Dr Bhupal Dev of Washington State University, axions and axion-like particles (ALPs) could be created within the extreme conditions of a neutron star collision, and we might be able to see their signature from Earth.
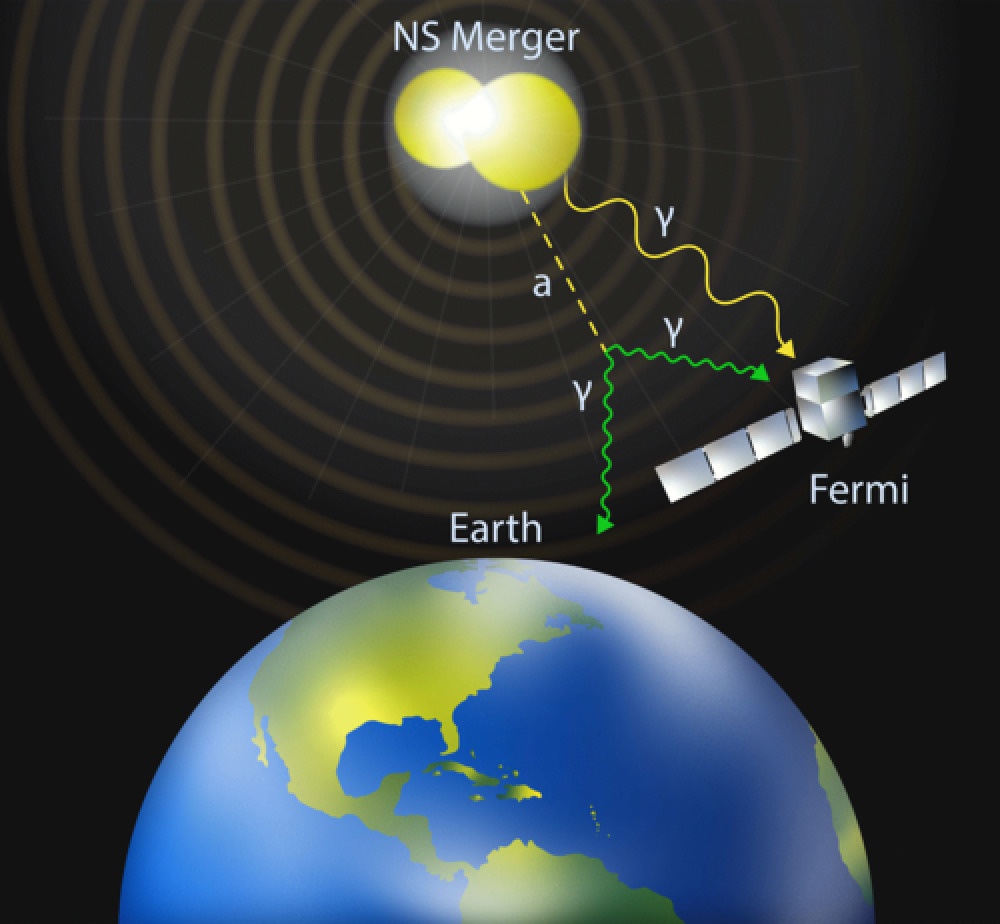
Physicists have spent decades trying to solve the mystery of dark matter. It seems likely that it could be made mostly from axions and axion-like particles, but these particles are still only hypothetical. The axion was first proposed in 1977, as a solution to the Strong CP Problem, but has yet to be confirmed.
Theory predicts, however, that axions can be briefly created by passing high-energy photons through a powerful magnetic field. These axions last for a short while, then decay back into a pair of gamma-ray photons. A number of experiments are being conducted around the world, using this phenomenon to try and create axions, and watching for the gamma radiation of their decay. Others, like the Axion Dark Matter eXperiment (ADMX) are looking for naturally existing axions by using a similar process to convert them into microwave photons.
But there are lots of places in the Universe where axions can be created in this manner, including the cores of stars, around magnetars, and anywhere else with strong magnetic fields. One possible location is the site of a neutron star collision. When such massively dense objects collide, they release a tremendous amount of energy, some of it in the form of hard electromagnetic radiation and powerful magnetic fields: perfect conditions to create axions!
By modelling the energies involved, researchers can predict the masses of axions that will be produced. From there they can deduce the specific frequency of gamma ray photons that would be produced when they decay. If we can detect another such merger, and spot that specific spectrum of gamma radiation coming from the collision, that would confirm that axions are real, and provide evidence supporting a major theory about dark matter.
Natural particle accelerators
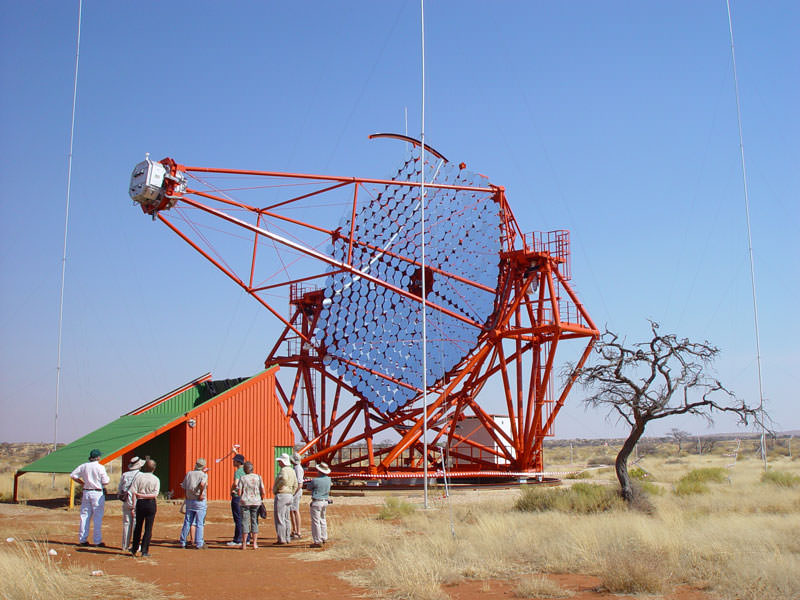
An experiment like this would not be the first time scientists have tried to use natural events in place of a particle accelerator. Our own upper atmosphere is one such place where high energy particle collisions happen all the time. Unlike gamma radiation, cosmic rays are subatomic particles hurtling through space at relativistic speeds, and they from catastrophic events like supernova explosions. When they encounter our atmosphere, they smash into air molecules with greater violence than we are able to create in our largest particle accelerators. Telescopes like the High Energy Stereoscopic System (HESS) in Namibia are built to detect these collisions, high up in the sky. HESS is a pair of telescopes which focus on the upper atmosphere, looking for the characteristic bursts of cherenkov radiation that reveal the cascades of particles generated whenever a cosmic ray smashes into the atmosphere.
The observations from GW170817 have already been used by Dr Dev: careful analysis of the gamma rays observed by Fermi-LAT have already helped to narrow the constraints on the properties of axions and axion-like particles.
Observations like this, combined with the work of earth-bound experiments like ADMX, are critical to finding out whether axions exist. And although they haven’t found it yet, we still learn something each time an experiment fails to find anything. Each test is tuned for a specific mass, so those negative results all work together to narrow the range of possibilities. Hopefully it won’t be long before we have a definitive answer.
To learn more, visit https://source.wustl.edu/2024/03/finding-new-physics-in-debris-from-colliding-neutron-stars/
Other axion searches on the table includes GALILEO.
“By measuring the differential phase velocity between the two arms of the interferometer, GALILEO can detect the frequency of oscillation induced by light dark matter [axion-like particles]. This oscillatory signal serves as the signature of the presence of dark matter particles.”
“”Our proposal uses similar technological advancements as LIGO, such as Fabry-Perot cavities or squeezed light to suppress the quantum noise limit. However, unlike LIGO, the proposed GALILEO interferometer is a tabletop-scale device.””
[“GALILEO: Scientists propose a new method to search for light dark matter”, by Tejasri Gururaj , Phys.org]
It may work, however they are not very compelling IMHO.
Cosmologically they are a bad fit to cold (heavy) dark matter. Quantum physically they have a gravitational coupling problem I believe (most of the potential mass range has too short lifetime to exist as primordial dark matter today). Finally standard particle CP symmetry breaking is better explained by its finetuning (CP symmetry; finetuning factor now in the minimum range of 1,000 – 1,000,000 without any axions involved) and neutrinos (mass oscillation CP breaking the putative axion symmetry now at 2 sigma significance).