If the Sun has a stellar neighbourhood, it can be usefully defined as a 20 parsec (65 light-years) sphere centred on our star. Astronomers have been actively cataloguing the stellar population in the neighbourhood for decades, but it hasn’t been easy since many stars are small and dim.
Even with all of the challenges inherent in the effort, astronomers have made steady progress. Do we now have a complete catalogue?
In a new article in Research Notes of the American Astronomical Society, a pair of researchers from the Leibniz Institute for Astrophysics in Potsdam, Germany, try to understand how complete or incomplete our catalogue of the stellar neighbourhood is. The article is titled “Do We Finally Know all Stellar and Substellar Neighbors within 10~pc of the Sun?” The authors are Ralf-Dieter Scholz and Alexey Mints.
If all stars shone as brightly as main sequence stars like our Sun do, it would be easy to catalogue the stars in our neighbourhood. But they don’t. Some are so small and dim that they’re considered failed stars. We call them brown dwarfs or substellar objects.
When we look up at the night sky with the unaided eye, our view is dominated by main sequence stars and giant stars, many of which are far beyond our stellar neighbourhood. Many stars are too dim to see, like red dwarfs and brown dwarfs. In fact, Proxima Centauri, a red dwarf and our nearest neighbour, wasn’t discovered until the early 20th century.
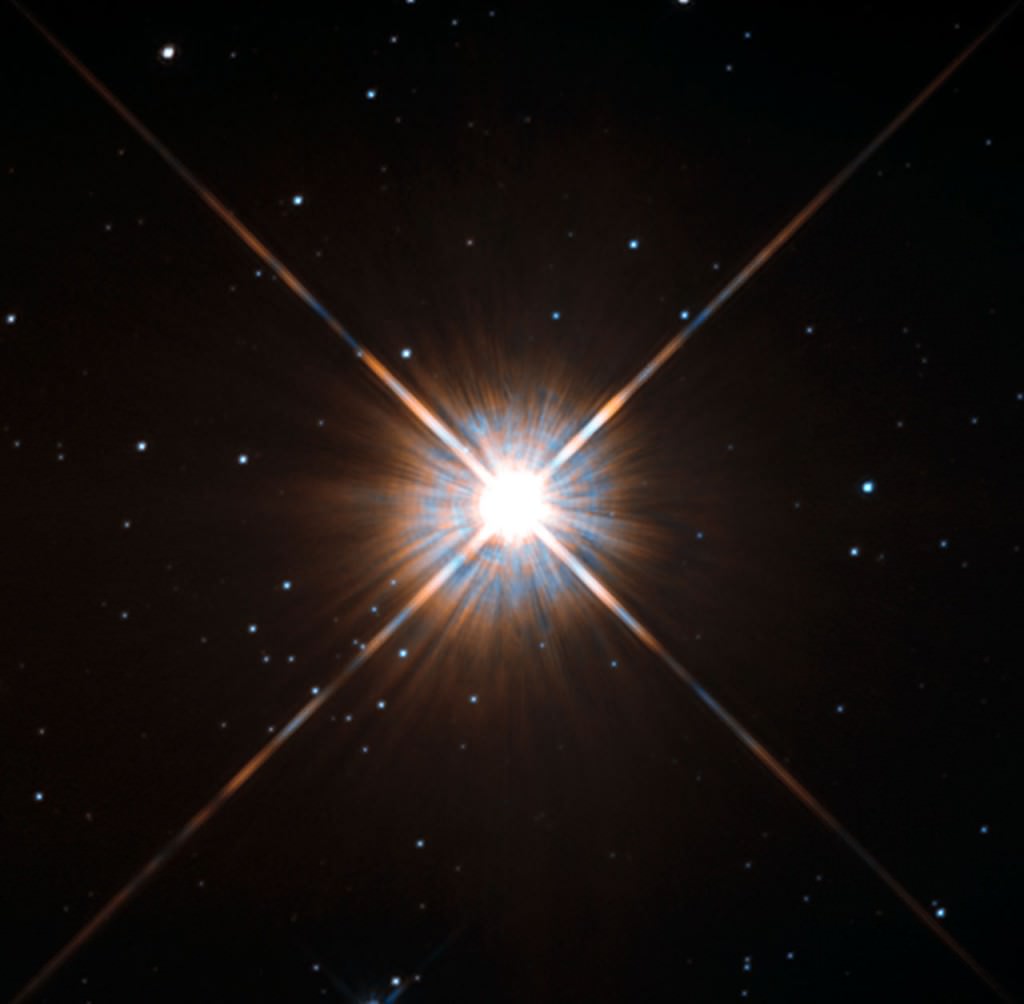
In the early days of astronomy, measurements of proper motions showed that some stars that appear fixed in place are closer than other stars. All stars move and have proper motion; it’s just not always noticeable in the span of a single lifetime. High proper motion surveys of stars led to the selection of certain stars for measurements of their parallax, which helped locate more stars correctly in space. Then, in the early 20th century, as astronomy and photography were used in conjunction, photographic astrometry triggered a wave of discoveries of our solar neighbours. Those efforts showed that our nearest neighbours are red dwarfs (M dwarfs).
In the 1990s, as technology advanced, infrared sky surveys found more dim stars. “A second wave of discoveries started in the late 1990s with the advance of infrared sky surveys,” the authors write. Missions like the Two Micron All-Sky Survey (2MASS) gave us a new, unprecedented look at the sky. It found M dwarfs, brown dwarfs, and substellar objects like L, T, and Y types, and even minor planets in the Solar System. (Definitions of brown dwarfs and other substellar objects overlap.) By the year 2,000, the Sloan Digital Sky Survey came online, strengthening our catalogue of the sky.
In 1997, Henry et al. published an important paper on the solar neighbourhood titled “The solar neighborhood IV: discovery of the twentieth nearest star.” It showed that the discovery of LHS 1565, about 3.7 pc from Earth, spelled trouble for our census of the neighbourhood. “It ranks as the twentieth closest stellar system and underscores the incompleteness of the nearby star sample, particularly for objects near the end of the main sequence,” Henry et al. wrote. “Ironically, this unassuming red dwarf provides a shocking reminder of how much we have yet to learn about even our nearest stellar neighbours.”
Since about 1997, there’s been a burst of discoveries of stars within the Sun’s neighbourhood. The authors say that these seem to have filled in the gaps in our 10 pc neighbourhood. But some of the knowledge was still based on two assumptions. The first was that the survey out to 5 parsecs was complete, and the second was that the density was uniform out to 10 parsecs. “The first of these is not true, and the second is in question,” the authors write.
Where does that leave us? Up to 90 star systems could still be missing.
![An artist's conception of a brown dwarf. A new study identifies CK Vulpeculae as the remnant of a collison between a brown dwarf and a white dwarf. Image: By NASA/JPL-Caltech (http://planetquest.jpl.nasa.gov/image/114) [Public domain], via Wikimedia Commons](https://www.universetoday.com/wp-content/uploads/2018/10/Artist’s_conception_of_a_brown_dwarf_like_2MASSJ22282889-431026-1024x576.jpg)
“Using all neighbours the luminosity and mass functions and the star-to-brown dwarf (BD) number ratio can be studied,” the authors state. Astronomers don’t fully understand the ratio of brown dwarfs to other stars, but two recent papers (1,2), in particular, have continued the work to better understand and catalogue our stellar neighbourhood’s dim members.
Earlier this year, Kirkpatrick et al. published a study claiming that a complete survey of nearby stars is possible, largely thanks to Gaia data. They found 462 objects (including the Sun) in 339 systems within 10?pc. of the Sun.
In previous work, the authors of this new paper added 16 more stars to the list. These were late M-dwarfs, some of the coolest and dimmest main sequence stars, and brown dwarfs. They also discovered a new white dwarf companion to an existing M dwarf.
But how complete is this newest survey?
The problem lies in the difficulty of detecting dim stars like brown dwarfs and late M-dwarfs. The further we look, the more difficult they are to detect. They’re also more difficult to detect in the direction of the galactic plane.

The authors say that our neighbourhood stellar catalogue is still likely missing 93 stellar systems, “… corresponding to a deficit of ?21.5%,” they write. In terms of individual stars, it’s not much better: “…138 missing objects corresponding to a deficit of ?23.0%,” they write.
They broke it down even further to individual star types. We’re probably missing 28.1% of AFGK stars, -31% of white dwarfs, and ?27.8% of M-dwarfs. There’s also a higher deficit for late M-dwarfs. These deficits are higher than expected. What does it mean?
“The estimated deficits of systems and individual objects within 10?pc exceed expectations, in particular for the well-known AFGK stars,” the authors write. They conclude that the general assumption of a constant stellar density in the solar neighbourhood is incorrect. They say that small-scale density fluctuations can at least partly explain the deficits.
“Our statistical estimates show that the probability of these discrepancies being caused by random fluctuations is around 40%,” the authors conclude.
We clearly have more work to do.