As it’s name suggests, dark matter is dark! That means it’s largely invisible to us and only detectable through its interaction with gravity. One of the leading theories to explain the stuff that makes up the majority of the matter in the Universe are WIMPs, Weakly Interacting Massive Particles. They are just theories though and none have been detected. An exciting new experiment called LUX-ZEPLIN has just completed 280 days of collecting data but still, no WIMPs have been detected above 9 Gev/c2. There are plans though to narrow the search.
The concept of dark matter was first proposed by Fritz Zwicky in 1930’s who noticed that galaxies were moving too fast to be held together by ‘normal’ visible matter. His work was expanded upon by Vera Rubin in the 1970’s who confirmed that stars around the outer regions of galaxies were moving faster than expected. The conclusion of these observations is that there was some form of invisible matter making up about 85% of the mass of the Universe. New results from LUX-ZEPLIN are homing in on the WIMP model to describe the nature of dark matter.

LUX-ZEPLIN is an instrument designed to detect dark matter. Located 1.6km underground at the Sanford Underground Research Facility in South Dakota, it’s a massive tank filled with 10 tons of liquid xenon waiting and watching for tiny interactions between dark matter and normal particles. It is shielded from cosmic radiation and other background noise by an onion layer design with each layer blocking out radiation or tracking particle interactions to rule out erroneous dark matter interactions. The principle is simple, a passing WIMP could knock into a xenon nucleus causing it to move and emit light and electrons in the process. It is these signals the teams are looking for. Along with new analytical techniques the teams using it hope they will finally unlock the mysteries of dark matter.
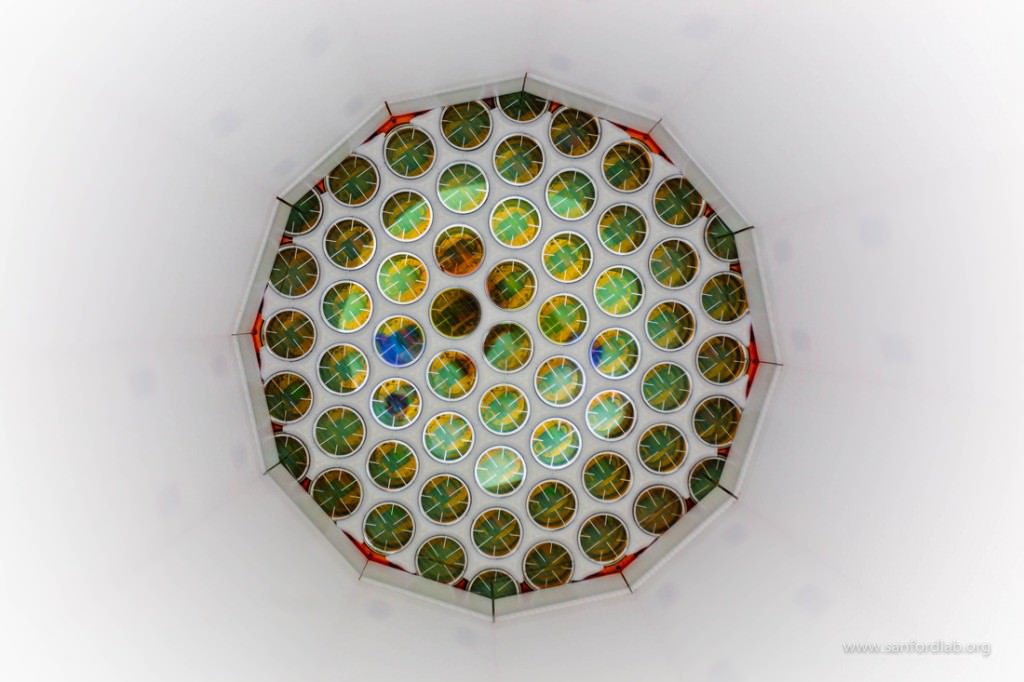
Following 280 days worth of data, no dark matter WIMPs were detected. Focussing their attention on WIMPs with a mass above 9 Gev/c2 the team found nothing despite the sensitivity of the detector. Having explored 9 Gev/c2 the team plans to start probing different energy levels as the hunt continues. The results had been announced at two physics conferences on 26 August TeV Particle Astrophysics 2024 in Chicago and LIDINE 2024 in São Paulo.
The team applied an interesting new technique called ‘salting’ which adds fake WIMP signals during the collection of the data. The approach hides real data until the very end when an ‘unsalting’ technique removes them. This avoids unconscious bias and stops researchers from overly interpreting the data.
Over the few years and by 2028, the team plan to collect at least 1,000 days of data which will be analysed using the new techniques. They will be using the data to study other rare phenomenon like the decay of xenon atoms, neutrino-less double beta decay and born-8 neutrinos from the Sun. There is still much work to do but the 250 scientists at LUX-ZEPLIN are hopeful. LUX-ZEPLIN’s physics coordinator Scott Kravitz from the University of Texas summed it up beautifully ‘Our ability to search for dark matter is improving at a rate faster than Moore’s Law. If you look at an exponential curve, everything before now is nothing. Just wait until you see what comes next.’
Source : Experiment sets new record in search for dark matter
If dark matter is just a thick soup of primordial cold neutrinos, how do we detect that?
I partly agree with kavalec, since the xenon experiments tend to stop at several GeV/c^2 they don’t probe the neutrino sector with the sterile WIMP candidates at 1-2 MeV/c^2. (Set by interactions with gravity and time to have galaxies, unless they are somehow protected like the Higgs sector.)
In that sense these experiments looks like the LEP experiment that could exclude Higgs masses just shy of the actual mass. I’m happy with massive supersymmetry WIMP exclusion here (and partly in LHC and electron sphericity experiments). But it is also frustrating, I wish they came up with experiments that directly target the least extended – so perhaps most likely – Standard Model candidate. Instead experiments target everything else, much lighter axion and much heavier supersymmetry candidates, both possible but less likely (as axions are now too light for cosmology and supersymmetry now too massive for accelerators).
@kavalec: An exclusive standard neutrino background has been excluded by e.g. Planck, standard neutrinos consist at most 0.2 % of dark matter.
There’s also this going for WIMPs: They may solve the final parsec problem of merging supermassive black holes (among other things).
“The simplest model for dark matter assumes that it is collisionless, which means that dark matter does not interact with itself. But there are some models of dark matter where it can weakly interact with itself. These models are known, appropriately enough, as self-interacting dark matter. Astronomers proposed this model of dark matter decades ago as a way to solve some of the deficiencies with the collisionless dark matter idea. Most importantly, collisionless dark matter has difficulty producing the observed number of dwarf satellite galaxies and matching the observed densities in the cores of galaxies. ”
“The astronomers behind the study found that when they switched out collisionless dark matter for self-interacting dark matter in their models, the final parsec problem wasn’t a problem anymore. Supermassive black holes are thought to merge as the last stage in the merger of two galaxies. As the supermassive black holes enter the core of the resulting single galaxy, they encounter greater densities of dark matter. The black holes interact with the dark matter through gravity — just as they do with gas and stars when they are farther apart — which siphons energy from the momentum of the black hole into the dark matter particles. With collisionless dark matter, the dark matter particles pick up this extra energy and then simply leave. But with self-interacting dark matter, the extra energy added to the particles just goes into more interactions.
This allows the dark matter to act as a reservoir that can absorb the kinetic energy of the black holes as they come closer together. With this extra reservoir available, the supermassive black holes quickly close the final parsec and meet in their final gravitational embrace.”
“How merging black holes could reveal the nature of dark matter”, Paul Sutter, Astronomy