[/caption]
It’s possible that if we do eventually observe the hypothetical objects that make up the hypothetical Oort cloud, they will all be a deep red color. This red coloring will probably be a mix of ices, richly laced with organic compounds – and may represent remnants of the primordial material from which the solar system was formed.
Furthermore, the wide range of colors found across different classes of trans-Neptunian objects may help to determine their origins.
The current observable classes of trans-Neptunian objects includes Pluto and similar objects called plutinos, which are caught in a 2:3 orbital resonance with Neptune towards the inner edge of the Kuiper belt. There are other Kuiper belt objects caught in a range of different resonant orbital ratios, including two-tinos – which are caught in a 1:2 resonance with Neptune – and which are found towards the outer edge of the Kuiper belt.
Otherwise, the majority of Kuiper belt objects (KBOs) are cubewanos (named after the first one discovered called QB1), which are also known as ‘classical’ KBOs. These are not obviously in orbital resonance with Neptune and their solar orbits are relatively circular and well outside Neptune’s orbit. There are two fairly distinct populations of cubewanos – those which have little inclination and those which are tilted more than 12 degrees away from the mean orbital plane of the solar system.
Beyond the Kuiper belt is the scattered disk – which contains objects with very eccentric elliptical orbits. So, although it may take hundreds of years for them to get there, the perihelions of many of these objects’ orbits are much closer to the Sun – suggesting this region is the main source of short period comets.
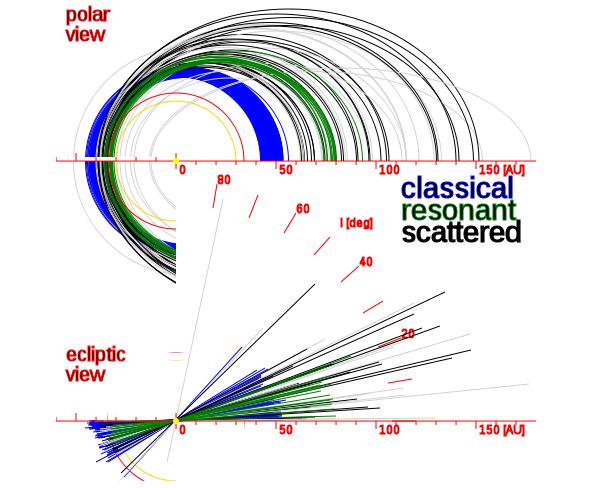
Now, there are an awful lot of trans-Neptunian objects out there and not all of them have been observed in detail, but surveys to date suggest the following trends:
- Cubewanos with little inclination or eccentricity are a deep red color; and
- Plutinos, scattered disk objects and highly inclined cubewanos are much less red.
Beyond the scattered disk are detached objects, that are clearly detached from the influence of the major planets. The best known example is Sedna – which is… yep, deep red (or ultra-red as the boffins prefer to say).
Sedna and other extreme outer trans-Neptunian objects are sometimes speculatively referred to as inner Oort cloud objects. So if we are willingly to assume that a few meager data points are representative of a wider (and hypothetical) population of Oort cloud objects – then maybe, like Sedna, they are all a deep red color.
And, looking back the other way, the ‘much less red’ color of highly inclined and highly eccentric trans-Neptunian objects is consistent with the color of comets, Centaurs (comets yet to be) and damocloids (comets that once were).
On this basis, it’s tempting to suggest that deep red is the color of primordial solar system material, but it’s a color that fades when exposed to moderate sunlight – something that seems to happen to objects that stray further inward than Neptune’s orbit. So maybe all those faded objects with inclined orbits used to exist much nearer to the Sun, but were flung outward during the early planetary migration maneuvers of the gas giants.
And the primordial red stuff? Maybe it’s frozen tholins – nitrogen-rich organic compounds produced by the irradiation of nitrogen and methane. And if this primordial red stuff has never been irradiated by our Sun, maybe it’s a remnant of the glowing dust cloud that was once our Sun’s stellar nursery.
Ah, what stories we can weave with scant data.
Further reading: Sheppard, S.S. The colors of extreme outer solar system objects.
There are a lot of reasons why bodies goes red in the solar system – Mars photo-oxidation/small grain weathering, solar radiation and micrometeoritic damage of asteroids, tholins on outer solar system bodies and the current pesky heat wave.
I’m not so sure about the bleaching though, alas “scant data” as mentioned. Mars and asteroids gets bleached by fresh material exposed.
The post mentions exposure to moderate sunlight for tholins, which doesn’t pin down the mechanism; photochemical bleaching (so what happens with tholins in the lab?), thermal alteration? The paper mentions thermal alteration specifically, but still doesn’t explain the mechanism.
You would think that it could be akin to Mars and asteroids getting fresh material exposed by letting go of volatiles, but apparently bodies like Sedna doesn’t have enough volatiles (nitrogen) for some of that. It’s a mystery.
Maybe the Tholins was formed by the winds and emission from one or more massive OB-stars in the small cluster expected to have birthed the Sun. Well, assuming that is how the Sun was birthed ofc.
At the fifth paragraph, in the third line:
Superfluous “these” there.
Interesting, but I fail to see the significance. What does it matter?
@ IVAN3MAN_AT_LARGE
Thanks – fixed.
@ billy_stanley
Agreed – that is generally my first thought upon waking (after coffee anyhow).
The whole point is these Kuyper belt planetoids or plutoids are frozen remnants of the early solar system. Maybe we will get some data from the Pluto flyby mission. I don’t know if we are going to get probes landed on Pluto or any of these bodies any time soon.
LC
What I want to know is why ever single render of any solar system object always shows one of three things:
1. Sun is a different brightness
2. Less or more stars/dust
3. Dimmer/brighter celestial body
For example: one render of Pluto will have a really bright sun, another a dim one, a render may have zero stars, or a few, or lots of stars and a brightly colorful galactic disk.
I understand artist like to take a creative license with these drawings… but when there seems to be little standardization to the look of “space” then how can I trust these artists to capture the celestial body?
Photographs taken in space of a planet or the shuttle over the Earth don’t capture star light. The much higher luminosity of this local light washes out starlight. However, if you were looking with eyes you would see star light.
For Pluto at about 20 AU out, the 1/r^2 decrease in light with radius r, means that Pluto would glow at about 1/400 that of moon light. So if you were looking at it up close with naked eyes it would dimmly glow. So to generate an image one must integrate or do a summation of light hitting a CCD or photoplate. Event still, as seen with Cassini images, the accumulation of photons from local objects would tend to obscure distant star light.
Since the sunlight out by Pluto is about 1/400 the luminosity on Earth, the sun would cast about as much light as the moon does here. Yet the light would appear concentrated on a small spot.
LC
LBC. You made correct use of the inverse square law, however Pluto is on average about 35 Au away from the sun. At this distance, the intensity of the light would be ~ 1/1200 of the intensity here on Earth.
Further, the full moon is on average only 1/10000 the brightness of the Sun. The perceived intensity of the moon is due to our eye’s ability to adapt to lower light conditions by dilating. Since this is almost 3 magnitudes brighter, we would have no trouble discerning even dark red and violet colors on Pluto.
Oops. It seems I left out a zero on my comment above. The full moon is less than 1/300000 the brightness of the sun. The actual values I can look up center around a magnitude 13.7 difference between the Sun and moon.
On Pluto, the current value for the Sun is ~ magnitude -19.7 which is only a 7 magnitude difference from the Sun (-26.7) This amounts to a brightness increase of 6 magnitudes for the Sun on Pluto as compared to the full moon on Earth.
I was working on memory with the numbers. I thought the moon was a few thousandths the radiant intensity of the sun, not 3e^{-5}. Of course luminosity is logarithmic, so a 6 difference is about what you quote.
LC
Our eyes do a lot of interesting things to cover such a large scale logarithmically.
IIRC, and this is really old data that I researched for a failed book chapter [not my idea, nor the EPIC FAIL!] so in need of update, there is a 10^3 range covered by adjusting the photosensitivity of rods and cones amplifying detected light. I.e. the output vary little with drastically increased input due to the mechanism of the pathway between the photosensitive compound, the membrane mechanism that integrate and amplify near simultaneous photon captures, and the final output to the neurons.
There is of course also a changeover/trade off between rods (b/w) and cones (color), as well as the usual neuronal net depression and recovery phases of strong signals that makes up for much of the rest of the controlled amplification.
That the eye can go from a lousy but yet functional single photon detector to day light function is amazing. But I envy cats that use a reflective layer to effectively double the chance of photon detection; and it makes for such pretty eyes too!
Looking into the sun quickly destroys our eyes, and our log3 eyes have to logarithmically adjust at night to see the full moon with the help of vitamin a and exposure time. It seems a cats eyes would fry sooner then our eyes looking at the sun, because their eyes are more advanced by having a relective layer to detect rare motion excited photons. Only by closing the eyelids and looking away and shielding from the source can intense eye radiation damage be prevented. I doubt a better eye can exist that can increase sensitivity to photons without increasing the damage done by radiation exposure? However in outer space a cat would see the faint light from the most distant places better then us but get more damage from cosmic rays that caused flashes to appear real to apollo astronauts.
Agree the lead image is implausible – particularly since it’s Sedna at nearly 90 AU distance. The whole idea of the article is that objects at this distance are not heated/lit sufficiently to have their surfaces modified – unlike say Pluto which forms a thin atmosphere around the perihelion of its orbit.
Nonetheless, it is a nice image – and it’s red.
Wow, my comment generated a lot of attention! Thanks guys for helping to break down what the eye sees at these distances!
eyes must first detect motions, before shadows of objects become discernable. light from a moving rabbit at night sends out entangled photons having strange trajectories. These single photons are difficult for us to detect, but a cat has a reflective lens that makes its eyes glow in the dark and can catch this action in the infrared region. This is why a cat is so fascinated by motion. They are analyzing with their senses trying to understand motion and black hole shadows that create matter. sometimes they become so real lifelike appearance a cat will leap and claw the spot your hand that moves the fake mouse.