[/caption]
A Japanese team of astronomers have reported a strong correlation between the metallicity of dusty protoplanetary disks and their longevity. From this finding they propose that low metallicity stars are much less likely to have planets, including gas giants, due to the shorter lifetime of their protoplanetary disks.
As you are probably aware, ‘metal’ is astronomy-speak for anything higher up the periodic table than hydrogen and helium. The Milky Way has a metallicity gradient – where metallicity drops markedly the further out you go. In the extreme outer galaxy, about 18 kiloparsecs out from the centre, the metallicity of stars is only 10% that of the Sun (which is about 8 kiloparsecs – or around 25,000 light years – out from the centre).
This study compared young star clusters within stellar nurseries with relatively high metallicity (like the Orion nebula) against more distant clusters in the outer galaxy within low metallicity nurseries (like Digel Cloud 2).
The study’s conclusions are based on the assumption that the radiation output of stars with dense protoplanetary disks will have an excess of near and mid-infra red wavelengths. This is largely because the star heats its surrounding protoplanetary disk, making the disk radiate in infra-red.
The research team used the 8.2 metre Subaru Telescope and a procedure called JHK photometry to identify a measure they called ‘disk fraction’, representing the density of the protoplanetary disk (as determined by the excess of infra red radiation). They also used another established mass-luminosity relation measure to determine the age of the clusters.
Graphing disk fraction over age for populations of Sun-equivalent metallicity stars versus populations of low metallicity stars in the outer galaxy suggests that the protoplanetary disks of those low metallicity stars disperse much quicker.
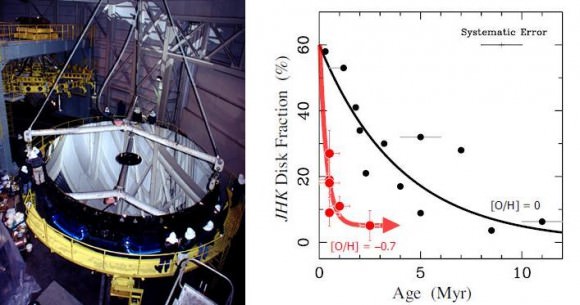
The authors suggest that the process of photoevaporation may underlie the shorter lifespan of low metal disks – where the impact of photons is sufficient to quickly disperse low atomic mass hydrogen and helium, while the presence of higher atomic weight metals may deflect those photons and hence sustain a protoplanetary disk over a longer period.
As the authors point out, the lower lifetime of low metallicity disks reduces the likelihood of planet formation. Although the authors steer clear of much more speculation, the implications of this relationship seem to be that, as well as expecting to find less planets around stars towards the outer edge of the galaxy – we might also expect to find less planets around any old Population II stars that would have also formed in environments of low metallicity.
Indeed, these findings suggest that planets, even gas giants, may have been exceedingly rare in the early universe – and have only become commonplace later in the universe’s evolution – after stellar nucleosynthesis processes had adequately seeded the cosmos with metals.
Further reading: Yasui, C., Kobayashi, N., Tokunaga, A., Saito, M. and Tokoku, C.
Short Lifetime of Protoplanetary Disks in Low-Metallicity Environments
That observation certainly can explain why the current exoplanet Fe/H data drops just below the sun’s metallicity to virtually zero below -0.5. (But not really, statistics being statistics, I can see a twitch in the -1 bin.)
Ah! Does that mean we still have a shot running at Galactic Overlords!?
There’s still a chance of those pesky black cloud hyper-intelligences.
Uhhhh….the included photo of the ‘Subaru Telescope’ looks like the *mirror* of the Subaru Telescope (probably in the process of being re-aluminized). This is the Subaru Telescope: http://www.subarutelescope.org/Introduction/telescope.html
Yes Jon, it’s the mirror – all 8.2 metres of it. Good call 🙂
For those actually interested in the periodic table see the excellent book,
Eric Scerri, The Periodic Table, Its Story and Its Significance, Oxford University Press, 2007. It traces the history and meaning of the periodic table as the title implies and much else besides, such as the relationship of the periodic table to quantum mechanics and the extent to which the latter has explained the former. Highly recommended reading.
http://www.oup.com/us/catalog/general/subject/Chemistry/?view=usa&ci=9780195305739
The early universe was predominantly H and He. Only a brief moment after the big-bang were conditions favorable for fusion of heavier elements. Planets didn’t come about until after the first early quasar supernovas ejected heavier elements, especially iron, which formed population 2 or 3 stars, like our sun and planet earth. Earths outer core 1,806 miles beneath the surface is a hot molten electrical conductive liquid having rotation and Dynamo effects producing a Magnetic Field in some ways similar to neutron stars. It comprises an astonishing 30.8% mass of the earth. Its composition is believed to be mostly molten iron, but 10% sulphur and oxygen dissolved, so that overall it is less dense then molten iron and contains lighter elements then the inner solid suspended nickel-iron core. Because inner terrestial planets are compositionally rich in alkali metals and iron especially earth like exoplanets, we can assume that the baby old 14.6 billion year universe that we live in will increase formation of earth like exoplanets for billions of years into the future as more supernovas occur. Eventually some say hydrogen will be depleted and fusion stars will fail and everything will go dark, until perhaps a few remaining infrared dwarfs fade into blackness.
Obviously there couldn’t be terrestrial or other rocky planets before stellar nucleosynthesis delivered the raw materials. The interest of this research is the proposal that you couldn’t have gas giants either.
The early metal poor universe perhaps tended towards massive stars with no planets – only when there were heavy elements were dust clouds then prone to fine structure fragmentation – making smaller long-lived stars more likely to form. And with heavier elements in their accretion disks, there was more likelihood of planet formation around those stars.
If all that’s correct, the probability of supernovae will decrease, rather than increase, going forward (due to the general tendency to form smaller stars as more metals accumulate).
Some say we live in a middle-aged universe.
I am tempted to say the reason for this is that heavier element have more degrees of freedom. There are more electrons in atoms to absorb and re-emit photons. As a result the protoplanetary disk of heavier element is more insulating, or acts as an insulator with respect to radiation indicence, and does not heat up as much for a given input of energy from the star. This would tend to mean a largely hydrogen gas would heat up more and expand away.
LC
LBC: I think you hit the nail right on the head with that. After reading this a largely came to the same conclusion you did. Even after less than a minute of thinking about it, it was easy to conclude that a dusty disk would be far more resilient to the radiation emitted by its host star than one consisting mainly of H-He.
What did surprise me was the implications it had for gas giants, but when I think about that for a minute or two longer, even that makes sense. Although the traditional gas giant is composed of mainly H-He (much like the early Universe), with no stable planetary disk present how can even those get going, even taking account the the relatively short time it takes to form them as proposed by the most commonly accepted theories? Gas giants need seeds to form, too.
Hey, now we’re cooking. Thanks Lawrence and Dave. I like the more electrons idea.
I have to confess that on the day after advancing this hypothesis I now have questions about it. For one thing, hydrogen has a low opacity to optical radiation. This means optical radiation interacts rather weakly with hydrogen. The problem with POPIII stars is in fact the stability problem, for a pure H-He gas does not thermalize readily with radiation it is difficult to understand the thermal properties required to get hydrodynamic equilibrium of these stars. This then suggests almost the opposite of what I proposed yesterday.
If I were to still uphold the degree of freedom aspect of the hypothesis, I have to modify something I should think. I would then say it might then be that heavier elements actually interact more strongly with the radiation (more degrees of freedom might support that) and as such would then amplify small inhomogeneous distributions in the protoplanetary disk.
LC
@ LC
I’m not sure these things need to be mutually exclusive. Heavy elements scatter light because they are relatively opaque – and perhaps get hotter in the process. Light elements just get blown away/dispersed. From the photoevaporation link: “The lower the mass number of the gas, the higher the velocity obtained by interaction with a photon. Thus hydrogen is the gas which is most prone to photoevaporation”.
I am not a solar system evolution maven, but I do remember a somewhat “hand waving” argument about rocky planets being close to the parent star for this reason. So in analogy with a metal that has largely free electrons in its conduction band as it thermal degree of freedom, hydrogen with few degrees of freedom and lightness is also rapidly heated up and “evaporated.”
LC
Inner planets contain metals that condense solidify at very high melting and boiling points. Gases are blown away from the protostar, helping form massive outer gas giants. Hydrogen can be a superconductor, superfluid, and supersolid ! Magnetic fields will phase change Hydrogen from a Superfluid to a Superconducter and vice versa. Interstellar space inside a galaxy is ~ 4 deg Kelvin, so H is a gas. larger black holes have colder temperatures increasing with mass radii ultimately would reach cool enough temperatures to make hydrogen gas form into a supersolid or superfluid or superconductor. This would prevade the medium of outer space at vast distances. the dark flow is a black hole at 150 billion light years that is so large, that everything for at least 2 billion light years is being steadily pulled towards that direction. the dark flow does NOT decrease with distance as Gravity does and I suggest that there could be solid or liquid hydrogen surrounding the black hole.