[/caption]
With so much of our current understanding of the universe based on Type 1a supernovae data, a good deal of current research is focused upon just how standard these supposed standard candles are. To date, the weight of analysis seems reassuring – apart from a few outliers, the supernovae do all seem very standard and predictable.
However, some researchers have come at this issue from a different perspective by considering the characteristics of the progenitor stars that produce Type 1a supernovae. We know very little about these stars. Sure, they are white dwarfs that explode after accumulating extra mass – but just how this outcome is reached remains a mystery.
Indeed, the final stages preceding an explosion have never been definitively observed and we cannot readily point to any stars as likely candidates on a pathway towards Type Ia-ness. In comparison, identifying stars that are expected to explode as core collapse supernovae (Types Ib, Ic or II) is easy – core collapse should be the destiny of any star bigger than 9 solar masses.
Popular theory has it that a Type 1a progenitor is a white dwarf star in a binary system that draws material off its binary companion until the white dwarf reaches the Chandrasekhar limit of 1.4 solar masses. As the already compressed mass of predominantly carbon and oxygen is compressed further, carbon fusion is rapidly initiated throughout the star. This is such an energetic process that the comparatively small star’s self-gravity cannot contain it – and the star blows itself to bits.
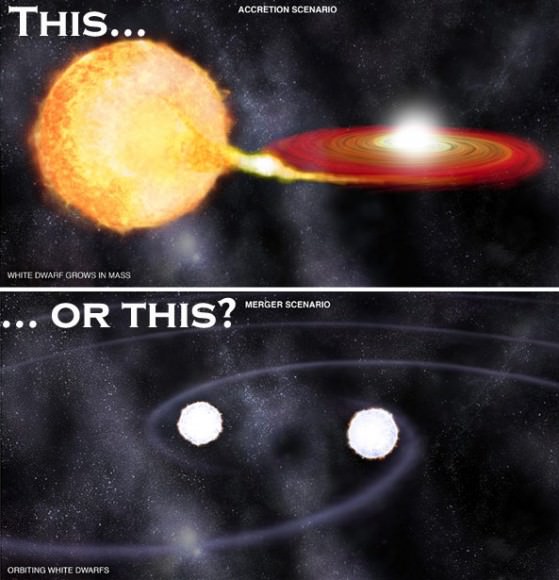
But when you try to model the processes leading up to a white dwarf achieving 1.4 solar masses, it seems to require a lot of ‘fine tuning’. The rate of accretion of extra mass has to be just right – too fast a flow will result in a red giant scenario. This is because adding extra mass quickly will give the star enough self-gravity so that it can partially contain the fusion energy – meaning that it will expand rather than explode.
Theorists get around this problem by proposing that a stellar wind arising from the white dwarf moderates the rate of infalling material. This sounds promising, although to date studies of Type 1a remnant material have found no evidence of the dispersed ions that would be expected from a pre-existing stellar wind.
Furthermore, a Type 1a explosion within a binary should have a substantial impact on its companion star. But all searches for candidate surviving companions – which would presumably possess anomalous characteristics of velocity, rotation, composition or appearance – have been inconclusive to date.
An alternative model for the events that lead up to a Type 1a are that two white dwarfs are drawn together, inexorably inspiralling until one or the other achieves 1.4 solar masses. This is not a traditionally favoured model as the time required for two such comparatively small stars to inspiral and merge could be billions of years.
However, Maoz and Mannucci review recent attempts to model the rate of Type 1a supernovae within a set volume of space and then align this with the expected frequency of different progenitor scenarios. Assuming that between 3 to 10 % of all 3-8 solar mass stars eventually explode as Type 1a supernovae – this rate does favour the ‘when white dwarfs collide’ model over the ‘white dwarf in a binary’ model.
There is no immediate concern that this alternate formation process would affect the ‘standardness’ of a Type 1a explosion – it’s just not the finding that most people were expecting.
Further reading:
Maoz and Mannucci Type-Ia supernova rates and the progenitor problem. A review.
Personnally and terribly unscientifically, I don’t see either scenario being the final outcome when we finally spot one in action. We would have spotted more “pre” scenes of white dwarfs gathering matter in the accretion scenario as the explosions are the rare event, but many would have to be setting up for fusion. The lack of companions is also very telling. The two white dwarfs conveniently supples the needed interaction and outcome but again, where are all the binary white dwarf systems? And why would “one or the other” gather 1.4 solar masses? Is that referring to when they bump?
Either bump or begin exchanging material as they get closer – I don’t know if anyone has outlined the process to this level of detail. I would think the latter is more likely to avoid the red giant scenario.
I guess the dwarf with the stronger surface gravity gets to 1.4 solar masses first and then kabloowie.
There are white dwarf binaries out there – e.g. this one which seems not far off merger:
http://www.sciencedaily.com/releases/2010/03/100309091450.htm
I would agree a lack of shell-shocked post-binary white dwarfs is a weakness of the argument. The article focuses on the estimated rate of SNe1a as its key point.
“kabloowie” – One of my favorite scientific terms! LOL!
The two mechanisms probably occur in the onset of a supernova. The accretion of matter from a companion star is probably the most reliable standard candle. The reason is that the Chandrasekar limit is reached slowly or adiabatically. The initial conditions for the implosion are then likely to be very similar and the energetic output of these stars very comparable. The collision of two white dwarf stars is likely to be much more variable.
It might then be important to discern the two mechanisms by observing signatures from the supernovae. There might be differences in the spectroscopy of the two.
LC
We do spot “pre” scenes of both types, the classical Nova that happens about a dozen times in our galaxy each year, ARE one of the possible “pre” scenes.
Classical Nova are white dwarfs accreting hydrogen rich matter from a nearby companion (usually a lower mass star in the late lifecycle = the red giant phase), wich semi-regularily ignites explosively and causes a Nova. The open question is if the accretion becomes larger than the matter ejected in the Nova explosion. If the ejection is smaller than the accretion, the Chandrasekhar limit is within range, and the recurring Nova might go out in a final blast.
White dwarf binaries are very hard to detect because of the properties of white dwarfs, and it is very likely there are many-many more to discover in our galaxy alone.
Those two types do seem to be the only two possible types at this time, if you have reason for some other type to exist please explain.
Sure, we all know the “classical” scenario but as yet, that is just the theory and it’s not without its holes. Your statement “classical nova…are…”pre”scenes” makes no sense. Nova are of course, “post” scenes. After the fact, the end result. Not nearly as indicative of its progenitor as we might like. I’m just saying that science always oversimplifies until the facts are learned. I think we’ll find out its a more fascinating answer than we currently imagine. Maybe when we find those elusive gravity waves we’ll spot some more binary dwarf pairs.
What I meant is that the Classical Nova likely is a pre-scene for one of the possible types of Sn Ia.
What I meant is that the Classical Nova likely is a pre-scene for one of the possible types of Sn Ia.
Quoting:
http://www.universetoday.com/85167/what-triggers-a-type-ia-supernova-chandra-finds-new-evidence/
There are a couple of popular theories: one scenario for Type Ia supernovas involves the merger of two white dwarfs. In this case, no companion star or evidence for material blasted off a companion should exist. In the other theory, a white dwarf pulls material from a “normal,” or Sun-like, companion star until a thermonuclear explosion occurs.
…
Both scenarios may actually occur under different conditions, but the latest Chandra result from Tycho supports the latter one.
…
“This stripped stellar material was the missing piece of the puzzle for arguing that Tycho’s supernova was triggered in a binary with a normal stellar companion,” said Fangjun Lu of the Institute of High Energy Physics, Chinese Academy of Sciences in Beijing. “We now seem to have found this piece.”
–end quote–
I would posit that either accretion or disks of spinning plasma involved in the accretion process or a combination of both could be involved in the syphoning of gas from the secondary star.
I was speculating in my posts that this process could also occur with two dense binary neutron stars / objects – assuming that the secondary may have been a red giant that transitioned to a neutron star as both objects spiral towards one another in a gravitational dance.
So what I find interesting is there could be boundry and class-crossing merger scenarios quite easily if we consider a combination of accretion, dual core-collapse, di-polar and duo-dipolar electromagnetic vortexes and superchanged plasma rings colliding/merging. These processes augur well for the creation of so called ‘stripes’ in the supernova blast remnant.
quoting:
http://en.wikipedia.org/wiki/Stellar_evolution
The energy transferred from collapse of the core to rebounding material not only generates heavy elements, but (by a mechanism which is not fully understood) provides for their acceleration well beyond escape velocity, thus causing a Type Ib, Type Ic, or Type II supernova. Note that current understanding of this energy transfer is still not satisfactory; although current computer models of Type Ib, Type Ic, and Type II supernovae account for part of the energy transfer, they are not able to account for enough energy transfer to produce the observed ejection of material.[9] Some evidence gained from analysis of the mass and orbital parameters of binary neutron stars (which require two such supernovae) hints that the collapse of an oxygen-neon-magnesium core may produce a supernova that differs observably (in ways other than size) from a supernova produced by the collapse of an iron core.[10]
The most massive stars may be completely destroyed by a supernova with an energy greatly exceeding its gravitational binding energy. This rare event, caused by pair-instability, leaves behind no black hole remnant.[11]
So to sum it up: In the case of binary X-ray objects, a tiger can change its stripes (and then explode magnificently or release prodigious energy).
Further reading:
http://en.wikipedia.org/wiki/Supernova
Several types of supernovae exist. Types I and II can be triggered in one of two ways, either turning off or suddenly turning on the production of energy through nuclear fusion. After the core of an aging massive star ceases generating energy from nuclear fusion, it may undergo sudden gravitational collapse into a neutron star or black hole, releasing gravitational potential energy that heats and expels the star’s outer layers. Alternatively a white dwarf star may accumulate sufficient material from a stellar companion (either through accretion or via a merger) to raise its core temperature enough to ignite carbon fusion, at which point it undergoes runaway nuclear fusion, completely disrupting it. Stellar cores whose furnaces have permanently gone out collapse when their masses exceed the Chandrasekhar limit, while accreting white dwarfs ignite as they approach this limit (roughly 1.38[7] times the solar mass). White dwarfs are also subject to a different, much smaller type of thermonuclear explosion fueled by hydrogen on their surfaces called a nova. Solitary stars with a mass below approximately 9 solar masses, such as the Sun, evolve into white dwarfs without ever becoming supernovae.
Although no supernova has been observed in the Milky Way since 1604, supernovae remnants indicate on average the event occurs about once every 50 years in the Milky Way.[8] They play a significant role in enriching the interstellar medium with higher mass elements.[9] Furthermore, the expanding shock waves from supernova explosions can trigger the formation of new stars.[10][11][12]
The differentiation of supernovae types is currently well defined with respect to the presence of hydrogen in the supernovae spectra.
Type Is (a,b & c) have virtually no hydrogen, while Type II’s do. I can’t immediately see how you could switch from one to the other – either there is pre-existing hydrogen, or there isn’t.
The types of supernova are based on spectra, length of spectral lines and the time profile of the luminosity measured. It is hard for me to always keep this straight. In the case here where the white dwarf slowly accretes material to its Chandrasekhar limit strikes me as the “perfect” standard candle. The physical conditions of such supernova should be nearly identical in all cases. The only possible difference might be the distribution of chemical elements, but in general we expect a strong carbon cycle. For colliding white dwarfs the Chandrasekhar limit is reached abruptly, and for two white dwarfs with a combined mass above M_{ch} = 1.4M_{sol} this can be a sudden jump to a much larger mass than M_ch. In fact it could be as much as twice that.
I am not that knowledgeable on supernova physics, but I should expect that there would be marked differences in the optical or electromagnetic characteristics measured from these two processes. I am not terribly educated on how such phenomenology would be, or has been, performed. Yet this does seem important for understanding better the tolerances on these cosmological standard candles.
LC
Thank you Steve and Lawrence.
Good point regarding pre-existing hydrogen and something I wasn’t aware of…
I am still trying to untangle (or re-tangle?) all this in my own reasoning.
I located a free peer-reviewed paper on the subject of Supernovae, Jets & Collapsars if other forum members are interested to read:
http://iopscience.iop.org/0004-637X/550/1/410/
Thanks again.
Wezley
Here’s my two cents, think of a supernovae as mass reaching and passing the critical weight of mass, much like when a sonic boom is heard when an object reaches and passes the speed of sound. so in essence, what we see is all the matter that has not yet reached this critical mass being blown off as heavier matter passes its own gravitational bow shock.
That makes very little sense when concerning type Ia supernovas, or (if true) pair-instability supernovas, as they are expected to completely obliterate themselves with no central remnant.
Core collapse supernovas is another matter, is that what you are referring to?
Wow Steve – I don’t know how you do it. My mind has been a churning cauldron ever since the good & kind folks at UniverseToday.com published:
http://www.universetoday.com/88996/steller-neutron-star-blows-away-model/#more-88996
and this
http://www.universetoday.com/91174/are-pulsars-giant-permanent-magnets/
and this
http://www.universetoday.com/91108/astronomers-complete-the-puzzle-of-black-hole-description/
and (I will make this the last but none the less of the highest calibre as with the previous articles):
http://www.universetoday.com/90917/different-supernovae-different-neutron-stars/
So it is fair to say X-Ray Binary Neutron Stars as a category and class are being re-dfined as a class of object – and the reportage of universetoday.com is a part of the action and history-making.
// Wezley Jackson Observation & Synthesis of above articles
These are immensly diverse objects encompasing but not limited to: Millisecond Pulsars, Black Holes, X-Ray binary neutron stars, Magnetars, Collapsars, Type 1A Super-Nova event, and there are some strange suggestion there could be 1A/II boundry crossers & early AGN with similar behavior but I don’t want to speculate.
I can imagine pin-wheel like gas strands accelerating towards relativistic speed. In this process of infall towards the host neutron star, the swirling knots detach and become ever increasingly fast and dense rings of charged electromagenic plasma in a high state of flux. At some threshold if there is enough matter infallling at a high enough velocity & a ring collision occurs, type IA can be trigerred – This would explain why the event is so hard to observe – It would be a very fast process triggered by a specific phase transition – I believe involving 10+ solar mass well above chandrasekhar limit.
The final piece of the puzzle, I believe could be due to two rings (one on a primary neutrons star and one on the secondary star) slamming into eachother during the accretion proccess onto the host star.
This of course is all due to the immense density and newly discovered vortexes of electromagetism generated by the (generally primary) neutron star.
Di-polar and Duo-Dipolar electromagnic fields & gravity waves are the new terms off the day encountered in these objects; In some small way this might be my one shot as an armchair cosmologist as having described roughly – I am sure the papers will come out and do a proper job.. I wish I finished that Degree darn it 😉
// end synthesis
Finding the articles above I realised the immense volume of high quality astrophysics articles being published by universetoday.com. Keep up the great work.
Wezley Jackson
Thanks Wezley, I agree we collectively kick a good deal of astronomical ass. It’s a privilege to mix with such esteemed company.
It’s really great and you guys are doing great job.. nice collection..truly i inspired from this write up, thanks a lot for sharing this information here..