[/caption]
The mysterious asteroid Vesta may well have more surprises in store. Despite past observations that Vesta would be nearly bone dry, newly published research indicates that about half of the giant asteroid is sufficiently cold and dark enough that water ice could theoretically exist below the battered surface.
Scientists working at NASA’s Goddard Space Flight Center in Greenbelt, Md., and the University of Maryland have derived the first models of Vesta’s average global temperatures and illumination by the Sun based on data obtained from the Hubble Space Telescope.
“Near the north and south poles, the conditions appear to be favorable for water ice to exist beneath the surface,” says Timothy Stubbs of NASA’s Goddard Space Flight Center in Greenbelt, Md., and the University of Maryland, Baltimore County. The research by Timothy Stubbs and Yongli Wang, of the Goddard Planetary Heliophysics Institute at the University of Maryland, was published in the January 2012 issue of the journal Icarus.
If any water lurks beneath Vesta, it would most likely exist at least 10 feet (3 meters) below the North and South poles because the models predict that the poles are the coldest regions on the giant asteroid and the equatorial regions are too warm.
This global map of average surface temperature shows the warmer equatorial zone of the giant asteroid Vesta is likely too warm to sustain water ice below the surface. But roughly half of Vesta is so cold and receives so little sunlight on average that water ice could have survived there for billions of years. The dividing lines (solid gray) are found at about 27 degrees north latitude and 27 degrees south latitude. This map, with temperatures given in kelvins, comes from the first published models of the average global temperature and illumination conditions on Vesta. Credit: NASA/GSFC/UMBC
If proven, the existence of water ice at Vesta would have vast implications for the formation and evolution of the tiny body and upend current theories.
The surface of Vesta is not cold enough for ice to survive all the time because unlike the Moon, it probably does not have any significant permanently shadowed craters where water ice could stay frozen on the surface indefinitely.
Even the huge 300 mile diameter (480-kilometer) crater at the South Pole is not a good candidate for water ice because Vesta is tilted 27 degrees on its axis, a bit more than Earth’s tilt of 23 degrees.
By contrast, the Moon is only tilted 1.5 degrees and possesses many permanently shadowed craters. NASA’s LCROSS impact mission proved that water ice exists inside permanently shadowed lunar craters.
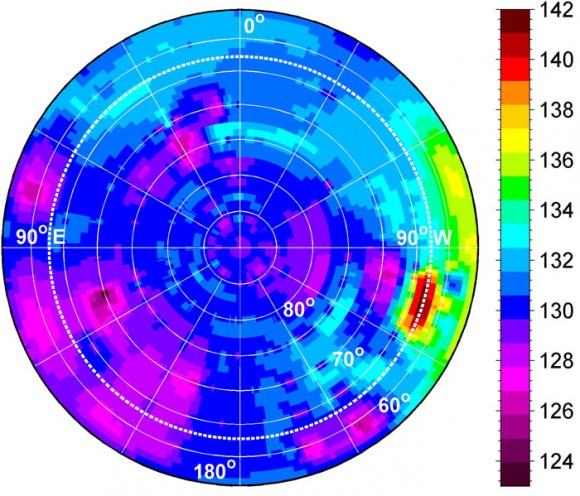
The models predict that the average annual temperature around Vesta’s poles is below minus 200 degrees Fahrenheit (145 kelvins). Water ice is not stable above that temperature in the top 10 feet of Vestan soil, or regolith.
At the equator and in a band stretching to about 27 degrees north and south in latitude, the average annual temperature is about minus 190 degrees Fahrenheit (145 kelvins), which is too high for the ice to survive.
“On average, it’s colder at Vesta’s poles than near its equator, so in that sense, they are good places to sustain water ice,” says Stubbs in a NASA statement. “But they also see sunlight for long periods of time during the summer seasons, which isn’t so good for sustaining ice. So if water ice exists in those regions, it may be buried beneath a relatively deep layer of dry regolith.”
Vesta is the second most massive asteroid in the main Asteroid belt between Mars and Jupiter.
NASA’s Dawn Asteroid Orbiter is the very first mission to Vesta and achieved orbit in July 2011 for a 1 year long mission.
Dawn is currently circling Vesta at its lowest planned orbit. The three science instruments are snapping pictures and the spectrometers are collecting data on the elemental and mineralogical composition of Vesta.
The onboard GRaND spectrometer in particular could shed light on the question of whether water ice exists at Vesta.
So far no water has been detected, but the best data is yet to come.
In July 2012, Dawn fires up its ion thrusters and spirals out of orbit to begin the journey to Ceres, the largest asteroid of them all.
Ceres is believed to harbor huge caches of water, either as ice or in the form of oceans and is a potential habitat for life.
If I remember the paper correctly that modeled the creeping liquid water zone on small differentiated bodies, anything below ~ 100 km wouldn’t be a good candidate for life, the zone moves too fast as the body cools down.
Presumably bodies that could have had or still have life includes Vesta and Ceres.
Wow, I’d like to see the “potentiality” for life to have evolved on a bit of powdery dust like Vesta. Life has so many needs here on Earth ie: light, air, warmth, food, condusive environments etc. hardly any of which are present on an airless, frozen mass like our second biggest asteroid. Hmm, “If proven, the existence of water ice at Vesta would have vast implications for the formation and evolution of the tiny body and upend current theories.” Since water is pretty much ubiquitous throughout the system (leaving out crispy Mercury and hot house Venus) I don’t see why water could not have factored in to the formation of asteroids. Clearly, they’ve seen their share of impacts some of which were probably comet derived.
“Life has so many needs here on Earth ie: light, air, warmth, food, condusive environments etc.”
Life on Earth needs only water, an energy gradient, and some kind of organic chemistry. Light, air, warmth, “food” as in parts of other organisms, they are not needed. Life is found in every location where you have water, energy, and organic chemistry. Many bodies in the Solar System have water and organic chemistry. The only reason why we have not found life yet is that we are very slow to look for it and spend far less than 1 percent of human productivity exploring space.
Hm, you say warmth is not needed but an “energy gradient” is. Wouldn’t that be the same thing? Light is also part of an energy gradient. I don’t think life started in the midst of a solid rock and all experiments to do with abiogenesis have assumed likewise. Life, having already begun, may be able to live in some extreme places or adapt over generations to them. However, I don’t think extremophiles would be likely precursors to our species. I would love to find life of any sort out there but we have yet to see any indications of such after having entered and investigated the alleged biospheres of at least five planetary bodies.
I am not sure what you mean by “warmth” here.
What is needed is as I said above, liquid water, organics and a redox potential. “Warmth” could be the temperature needed to hold water liquid. (This liquid could feasibly be replaced by other liquids elsewhere, say methane on Titan.)
Or you mean a temperature differential. That is helpful for abiogenesis (say, driving chemical flows in soup theories), but I don’t know of any extant life that manages to live on such alone.
The shorthand “energy gradient” isn’t really helpful to sort out current theories of abiogenesis. The hypothesis that anything else than redox potentials, which drives cellular metabolism, is enough is a hypothesis. It may be sound, it is in principle testable, but it is further removed than the parsimonious requirement of merely redox potentials, and also the idea that chemical evolution, most proficiently produced by redox potentials, seamlessly evolves into biological evolution.
That assumption isn’t actually the case.
The observation of catalysis and stereo-ordering, what positions atoms in molecules take, by mineral interfaces has been used by mainstream abiogenesis theories for a long time. Wäschterhäuser’s surface metabolism theory is from the 80’s, and Hazen et al have been extending that to a theory of abiogenesis in the crust at least from the 00’s.
You can also place Lane et al alkaline hydrothermal vent abiogenesis theories in that category, since they propose that the first cells were pores in the solid mineral “sponge” that forms around the vents. That habitable porous sponge is the direct equivalent to the asteroid porous habitable volume that Abramov et al discuss in the links I gave above, more so perhaps than Hazen’s crustal water-filled cracks. [I have now fixed the link fail.]
Hazen et al, as well as Lane et al, are currently investigating the respective environment abiogenesis potential, in situ (more so Hazen at a guess) and in experiments (more so Lane at a guess). To look down the martian crust, or as here large enough asteroids, would be an interesting and perhaps eventually necessary complement. The environments are pristine, so any extant biosphere or fossils from extinct life would be easier to recognize.
Mind that we are looking at different phenomena here, of potential abiogenesis, fossils of extinct life, and extant life. If Vesta’s chemical evolution got that far that we would call it life (reproducing populations) it would be interesting of itself.
Also, these different processes have different characteristics and environments. While abiogenesis is likely less robust than a mature biosphere with all its feedback processes, it is also fast and with little inherent ‘needs’.
Neither abiogenesis nor life would need “food” as it is part of the process. (Based on some redox potential, say light supplying plants which animals feed on.) Volatiles and temperature would be “condusive environments”.
Here is a description of the research on the conditions for asteroid life. (Paper here.)
“If it was 20% water, all of that water gets used up to make serpentine (or, to put it another way, water is the limiting reagent in the reaction), and the final asteroid winds up being dry and hot. With more than 20% water, there is water left over after the reactions have run their course, and because of water’s capacity to absorb heat, the resulting asteroid is cooler.”
“When an asteroid’s interior gets hot enough to melt water and that runaway serpentine reaction gets going, the center of the asteroid actually gets too hot; the Goldilocks zone is fairly close to the surface. As the asteroid cools off, the habitable zone migrates inward: regions closer to the center become cool enough as regions closer to the surface become too cold, freezing the ice. The cooling happens pretty fast, so the habitable zone migrates inward at rates of around 1 to 10 millimeters per year. (It happens more slowly for larger asteroids with more initial water.)
This is an important number, because recent research has shown that although asteroids can be very porous, the pores are tiny (5 to 50 nanometers across) and not well-connected, so liquid water cannot migrate from pore to pore very fast, if at all. Water, and any nascent life floating in it, certainly couldn’t have moved fast enough to keep up with the migration of the habitable zone.”
“Big asteroids are a different story. Big, ice-rich bodies like Ceres, or the body whose destruction gave rise to the Themis family of asteroids, would have differentiated, separating into layers of liquid water above a rocky interior, solving the pore space problem. Their long-lasting internal heat would likely have kept those oceans liquid for millions or tens of millions of years; Ceres may even have retained an internal ocean right down to the present day.”
From the paper:
“Increasing asteroid diameter and initial ice content positively affect habitable potential. The best case is Run 10, a 200-km asteroid with a 40% initial ice content, which experienced habitable conditions in over 85% of its radius and a duration of habitable
conditions of over 24 Myr in the center.”
Vesta is way larger, and could possibly have had life in its center at one time.