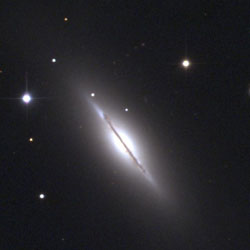
M102: “The Spindle Galaxy”. Image credit: NOAO/AURA/NSF . Click to enlarge.
Greetings, fellow SkyWatchers! This week begins with a meteor shower, but quickly turns galaxy hunt as we sail the galactic realm of the Virgo Cluster. If all you ask is a tall ship and a star to steer it by – then weigh anchor, because…
Here’s what’s up!
Tuesday, April 18 – Tonight let’s have a look at the Leo Trio – a superb group of two Messier galaxies and NGC 3628. Located some 35 million light years away, they make up their own smaller collection – the M66 galaxy group. All three may be framed together at low power and can best be located by first centering on Theta Leonis and sweeping a little more than a finger-width south to 73 Leonis. By putting star 73 less than a degree west, you will first see 9.5 magnitude M65 enter the low power field. M65 will soon be followed by brighter, larger and more face-on 9.0 magnitude M65. Both were discovered by Charles Messier on March 1, 1780. Larger and fainter yet is the irregular galaxy NGC 3628, which can be included in the low power field by shifting the pair south. Despite having a similar apparent magnitude, one look at this low surface brightness galaxy and you will easily forgive the famed comet hunter and his hard-working friend for missing it!
Want a challenge? Center your scope on 73 Leonis again and shift slightly more than half a degree southwest. Look for mid-sized 11th magnitude NGC 3593. Something even more difficult? How about 12th magnitude galaxy NGC 3596. This face-on spiral galaxy is also of low surface brightness and requires a large scope. Start at bright Chertan and shift less than a degree south-southeast to locate it.
Wednesday, April 19 – Tonight is an ideal time to study “Bode’s Galaxies” – now high in the northwest of the constellation of Ursa Major. To find this extraordinary pair of small scope studies, first locate Phecda (Beta) and 2 Dubhe (Alpha). Draw a line between this bright pair and extend that line an equal distance northwest beyond Alpha. Both galaxies are visible in large finderscopes or binoculars – but if you overextend, look for faint 24 Ursa Majoris and drop a finger-width southeast.
Discovered in December 1774 by J.E. Bode, these two deep sky favorites hold secrets between themselves. Photographed as early as March, 1899, the pair is central to a group of galaxies encompassing the northern circumpolar constellations Ursa Major and Camelopardalis. In small scopes and low powers, the two galaxies give the appearance of “Cat’s eyes” glowing in the night. Mid-sized scopes reveal the spiral nature of the brighter more southerly M81, while mottling can be seen in the irregular spindle shaped M82.
Center on M81 and make a shift of less than a degree southeast. This reveals two 8th magnitude stars forming a right triangle with 10th magnitude face-on spiral galaxy NGC 3077. More difficult is larger and fainter NGC 2976 – a tough find for even mid-aperture due to its low surface brightness and lack of a bright core. To locate the NGC 2976, return to M81 and shift the grand spiral slightly west about a degree and a half.
All four galaxies are part of the M81 group – a small galaxy cluster located some 12 million light years away. M81 and M82 are bound together in a powerful gravitational embrace. Only a few million years ago the two had a close encounter of a most difficult kind – one that largely devastated the structure of the less massive M82 but left its heavier companion completely intact with unrivaled spiral structure of great symmetry and beauty.
Thursday, April 20 – Are you ready to explore further? Then hold on to your star charts and get ready to be lost… The Coma Berenices galaxy group now enters the scene and there are so many galaxies visible that the hard part is being sure of what you are looking at! To get your bearings, start at Denebola (Beta Leonis) and move due east 6.5 degrees to the star 6 Coma Berenices. Once centered on 6 Comae, shift back toward Denebola a half degree to view one of the faintest of the Messier galaxies – M98 – a large near edge-on spiral. Discovered along with M99 and 100 by Pierre M?chain on March 15, 1781, the three discoveries became Messier’s last entries in the original published 3rd edition of his catalog. Although the view of 10th magnitude M98 may be disappointing through smaller scopes, this galaxy comes into its own using larger instruments where its nicely defined and expansive edge-on appearance becomes obvious.
By re-centering 6 Comae in the field and shifting less than one degree southeast, our next study – 9.8 magnitude spiral galaxy M99 – can be reliably identified. Should you go the same distance due east instead, you will encounter 11.5 magnitude NGC 4262. While due south this same distance you will find 11.2 magnitude NGC 4212 and its 13th magnitude neighbor – IC 3061. Although scopes can reveal M99 is a spiral today, it was Lord Rosse (in the spring of 1846) who first recognized the spiral nature of some galaxies.
M100, last on the originally published list, is found by again centering on 6 Comae. At low power, move 2 degrees northeast along a line of finder scope stars. M100 – at magnitude 9.4 – appears no brighter on the surface than M99 due to its greater apparent size. Like M99, M100 was included in Lord Rosse’s original 1850’s list of 14 spiral nebulae. Although face-on in presentation, M100 has two widely displaced and asymmetric spiral arms that can be detected visually through large scopes. Observers using mid-sized scopes should also look for 11.8 magnitude NGC 4312 south of M100. It’s also possible to see 13th magnitude IC 783 roughly that same distance due west.
Friday, April 21 – With dark sky to spare, tonight we continue our explorations of the Coma Berenices galaxy group – part of the larger Virgo supercluster of galaxies lying perpendicular to the plane of our own Milky Way galaxy.
Begin by first centering 6 Comae in the finder, then shift north-northeast 3 degrees to the disparate double, 11 Comae. Shift a little more than a degree due east to one of the brighter Messier galaxies in the Virgo cluster – 9th magnitude M85. In photographs, M85 looks like a giant elliptical galaxy but it’s a lenticular spiral completely devoid of arm structure. Located some 60 million light years away, this luminous mass of stars is relatively free of dust and has a diameter of 125,000 light years. M85 is larger than our own Milky Way, and more densely packed with stars.
A breath west of M85 is tiny 11th magnitude NGC 4394 – an easy study in moderately sized scopes. Slightly more than a degree in the opposite direction is larger 11th magnitude NGC 4293 – another round galaxy but one having a brighter core.
Before you call it a night, take a look east. Brilliant Jupiter is now taking up a year-long residence in Libra. You’ll need to wait for the planet to rise higher in the later evening to get a good view.
Saturday, April 22 – Today celebrates the birthday of Sir Harold Jeffreys. Born in 1891, Jeffreys was an early astrogeophysicist and the first person to envision an Earth with a fluid core in its center. Jeffreys also helped improve our understanding of tidal friction, overall planetary structure, and the origins of the solar system.
Up before dawn? Then enjoy the peak of the Lyrid meteor shower! Since the radiant originates near Vega, improve your odds of spotting them when the constellation Lyra is as high as possible. The Lyrid stream comes from parent comet Thatcher and produces about 15 bright, long-lasting meteors per hour.
Plan tonight to head into the Coma-Virgo galaxy cluster for more challenges. This time we’ll approach from Vindemiatrix (Epsilon Virginis) and move west-northwest along a chain of bright galaxies in the direction of distant Denebola. We’ll start with “Messier-quality” NGC 4762 followed by the M60, M59 and M58. Ready to starhop?
Our first stop lies a little more than a finger width west-northwest of Vindemiatrix: NGC 4762 is a 10.2 magnitude edge-on galaxy with a nearby 10.6 magnitude neighbor, NGC 4762. Most scopes show reveal a faint, thick lens-shaped patch of light oriented north-south. Like dozens of other bright NGC studies, NGC 4762 could have been discovered by Messier and friends in the eighteenth century – but wasn’t!
Continuing west-northwest another finger width reveals M60 – one of the brightest (magnitude 8.8) Coma-Virgo cluster members. This mid-sized elliptical galaxy condenses toward a bright core and shares the field with a pair of nearby companions (11.4 magnitude NGC 4647 and 11.3 magnitude NGC 4638). A touch west-northwest of the M60 group is a fainter (9.8) flattened elliptical galaxy M59. A bit further west is 10.9 magnitude galaxy NGC 4606 – a faint spindle of luminosity. All five of these galaxies can fit into a single low power field of view and will appear roughly as a line of nebulous islands hopping east to west!
Returning slightly east to center again on M59, we shift the scope a degree slightly north and further west to 9.8 magnitude M58. This small, face-on barred spiral is an original discovery of Messier – who found it along with M59 and 60 – while following a comet in the spring of 1779. Unknown to Messier was that the galaxies designated M59 and M60 in his log had already been discovered 4 days earlier (on April 11) by Johann Gottfried Koehler while pursuing the same comet!
Sunday, April 23 – Up early? Then be aware that the Moon occults Uranus. Check IOTA for details! Pioneering quantum physicist Max Planck was born this day in 1858. In 1900, Plank developed the equation explaining the distribution of light emitted by a theoretical “blackbody.” (Planck’s equation describes the relationship between the temperature of a body that absorbs all radiation falling on it – regardless of wavelength – and the wavelength of light radiated from that same body.) Interestingly, almost all the light seen in the heavens originates as “blackbody radiation” from the surface gases of stars. And where does the “absorbed light” come from? Nuclear fusion and the type of light that is far too vibrant for the human eye to see…
In honor of this principle, let’s turn our telescopes on the combined light of trillions of stars as we continue our exploration of the Coma-Virgo realm of galaxies leading up to the light of M87!
To begin tonight’s galaxy hop, start at Nu and extend a line to equal magnitude Omicron Virginis. Continuing the distance between Nu and Omicron places NGC 4429 at the northeast edge of a low power field. Fainter NGC 4371 may be seen less than a degree away northwest of NGC 4429. At magnitude 10.2, NGC 4429 appears about as luminous as previously studied M98. This near edge-on galaxy shows wispy spiral extensions and a bright star-like core.
Now move 1.5 degrees north for bright (magnitude 8.6) giant elliptical galaxy M87 – capital of the Coma-Virgo galaxy cluster. Look also for its 11.2 magnitude companion NGC 4478. Long exposure photographs of M87 reveal this 120,000 light year diameter radiant globe of luminosity is an “all stars” phenomenon. No matter what direction you might observe this giant from, you’d get almost precisely the same view – it’s similar to a massive globular cluster! M87 has collected tens of thousands of globular clusters, numerous smaller galaxies, and converted almost all of its matter to stars – a galaxy with a total mass exceeding several trillion suns.
Once you’ve located M87 it’s time to turn east-southeast (towards Vindemiatrix) for M58, M59, and M60. West-northwest is the direction of the “twin lenticular galaxies” – M84 and 86 – with their own galactic “field of dreams” – a place where large scopes can frame as many as a dozen galaxies in a single one degree field. Just a finger-width north of M87, you can find 9.5 magnitude tilted spiral M88 – looking like a “distant cousin” to the Great Galaxy in Andromeda seen from 60,000 million light years away. Had enough? No? Then head less than a degree west of M88 to find 10.2 magnitude barred spiral M91 in the same low power field. Less than a finger-width south-southeast of M91 is 9.5 magnitude M90 – another tilted spiral and one of eight galaxies (beginning with M84) found and later added to Messier’s list on the same productive night of March 18, 1781 (which also included the M92 globular cluster in Hercules.) How’s that for a night out under the stars?
May all your journeys be at light speed… ~Tammy Plotnerwith Jeff Barbour.