Download our free “What’s Up 2006” ebook, with entries like this for every day of the year.
|
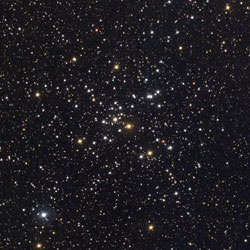
M41. Image credit: NOAO/AURA/NSF. Click to enlarge.
Monday, February 20 – Today in 1962, John Glenn was onboard Friendship 7 and became the first American to orbit the Earth. As Colonel Glenn looked out the window, he reported seeing “fireflies” glittering outside his Mercury space capsule. Let’s see if we can find some…
The open cluster M41 in Canis Major is just a quick drift south of the brightest star in the northern sky – Sirius. Even the smallest scopes and binoculars will reveal this rich group of mixed magnitude stars and fill the imagination with strange notions of reality. Through larger scopes, many faint groupings emerge as the star count rises to well over 100 members. Several stars of color – orange in particular – are also seen along with a number of doubles.
First noted telescopically by Giovanni Batista Hodierna in the mid-1500s, ancient texts indicate that Aristotle saw this naked-eye cluster some 1800 years earlier. Like other Hodierna discoveries, M41 was included on Messier’s list – along with even brighter clusters of antiquity such as Praesepe in Cancer and the Pleiades in Taurus.
Open cluster M41 is located 2300 light years away and recedes from us at 34km/sec – about the speed Venus moves around the Sun. M41 is a mature cluster, around 200 million years old and 25 light years in diameter. Remember M41…Fireflies in night skies.
Tuesday, February 21 – Be sure to have a look at the Moon this morning before dawn, because Jupiter will be joining it!
Tonight Luna will rise well after midnight, so let’s return to look at two of the few globular clusters of the season. Starting with M79 in Lepus, head due south around 15 degrees into Columba – the Dove. There you’ll find a second winter cluster almost a full magnitude brighter than M79 – NGC 1851. Give it a try!
Want another challenge? Head for bright Alnitak – the easternmost star in Orion’s belt. Using medium to low power, carefully shift bright Alnitak out from the center of the field about a full moon’s width to the west. With dark skies, you will see a large, faint, tulip-shaped nebulosity broken by one or more dark lanes. This is the “Flame Nebula”
– NGC 2024. Congratulations. This one isn’t easy, but on the darkest of nights it may surprise you!
Wednesday, February 22 – If skies are clear this evening, all you need do is step outside as the last glow of the long-set Sun pales to the southwest. Prepare your eyes – and heart – to follow the great expanse of the many brilliant stars of the winter Milky Way. Arching from Puppis to Cassiopeia, you might also see a fading Deneb – crown star of the Northern Cross – descending west. If you live towards the southern hemisphere, you should see brilliant Canopus – second brightest star in the night sky high to the south. In honor of the many splendid lights of the winter Milky Way, take out your binoculars and explore the marvels that await you!
Did you find something in the binoculars that caught your eye? Why not get the scope out and see if you can track it down. Navigating with a scope can be a challenge. Things look differently by eye, binoculars, finderscope, and telescope, but that’s what learning the night sky is all about.
Thursday, February 23 – On this date in 1987, Ian Shelton made an astonishing discovery – a supernova. At 160,000 light years away, distant SN1987a was the brightest novae display seen in almost 400 years. More importantly, before it occurred, a blue star of roughly 20 solar masses was already known to exist in that same location within the Large Magellanic Cloud. Catalogued as Sanduleak -69?202, that star is now gone. With available data on the star, astronomers were able to get a “before and after” look at one of the most extraordinary events in the universe! Tonight, let’s have a look at a similar event known as “Tycho’s Supernova.”
Located northwest of Kappa Cassiopeia, SN1572 appeared so bright in that year that it could be seen with the unaided eye for six months. Since its appearance was contrary to Ptolemaic theory, this change in the night sky now supported Copernicus’ views and heliocentric theory gained credence. We now recognize it as a strong radio source, but can it still be seen? There is a remnant left of this supernova, and it is challenging even with a large telescope. Look for thin, faint filaments that form an incomplete ring around 8 arc minutes across.
Friday, February 24 – In 1968, during a radio-telescope search for quasars, Susan Jocelyn Bell discovered the first pulsar. At first the regularity of the pulses was so precise that Bell and her college advisor, D. A. Hewish, thought they might be receiving a signal from a distant civilization. It soon became clear as the number of these objects multiplied that all were natural – rather than artificial – phenomena. Two co-directors of the project, Hewish and Ryle, later matched Bell’s observations to the notion of a rotating neutron star. This won them the 1974 Physics Nobel Prize and proved a theory brought forward thirty years earlier by J. Robert Oppenheimer.
Tonight let’s take a journey just a breath above Zeta Tauri and spend some quality time with a pulsar embedded in the most famous supernova remnant of all. Factually, we know the Crab Nebula to be the remains of an exploded star recorded by the Chinese in 1054. We know it to be a rapid expanding cloud of gas moving outward at a rate of 1,000 km per second, just as we understand there is a pulsar in the center. We also know it as first recorded by John Bevis in 1758, and then later cataloged as the beginning Messier object – penned by Charles himself some 27 years later to avoid confusion while searching for comets. We see it revealed beautifully in timed exposure photographs, its glory captured forever through the eye of the camera — but have you ever really taken the time to truly study M1?
Then you just may surprise yourself…
In a small telescope, M1 might seem to be a disappointment – but do not just glance at it and move on. There is a very strange quality to the light which reaches your eye, even though initially it may just appear as a vague, misty patch. Allow your eyes to adjust and M1 will appear to have “living” qualities – a sense of movement in something that should be motionless. The “Crab” holds true to many other spectroscopic studies. The concept of differing light waves crossing over one another and canceling each other out – with each trough and crest revealing differing details to the eye – is never more apparent than during study. To observe M1 is to at one moment see a “cloud” of nebulosity, the next a broad ribbon or filament, and at another a dark patch. When skies are stable you may see an embedded star, and it is possible to see six such stars.
Many observers have the ability to see spectral qualities, but they need to be developed. From ionization to polarization – our eye and brain are capable of seeing to the edge of infra-red and ultra-violet. Even a novice can see the effects of magnetism in the solar “Wilson Effect.” But what of the spinning neutron star at M1’s heart? We’ve known since 1969 that M1 produces a “visual” pulsar effect. About once every five minutes, changes occurring in the neutron star’s pulsation affect the amount of polarization, causing the light waves to sweep around like a giant “cosmic lighthouse” and flash across our eyes. M1 is much more than just another Messier. Capture it tonight!!
Saturday, February 25 – Since we’ve studied the “death” of a star, why not take the time tonight to discover the “birth” of one? Our journey will start by identifying Aldeberan (Alpha Tauri) and move northwest to bright Epsilon. Hop 1.8 degrees west and slightly to the north for an incredibly unusual variable star – T Tauri.
Discovered by J.R. Hind in October 1852, T Tauri and its accompanying nebula, NGC 1555, set the stage for discovery with a pre-main sequence variable star. Hind reported the nebula, but also noted that no catalog listed such an object in that position. His observations also included a 10th magnitude uncharted star and he surmised that the star in question was a variable. On each count Hind was right, and both were followed by astronomers for several years until they began to fade in 1861. By 1868, neither could be seen and it wasn’t until 1890 that the pair was re-discovered by E.E. Barnard and S.W. Burnham. Five years later? They vanished again.
T Tauri is the prototype of this particular class of variable stars and is itself totally unpredictable. In a period as short as a few weeks, it might move from magnitude 9 to 13 and other times remain constant for months on end. It is about equal to our own Sun in temperature and mass
– and its spectral signature is very similar to Sol’s chromosphere – but the resemblance ends there. T Tauri is a star in the initial stages of birth!
T Tauri are all pre-main sequence and are considered “proto-stars”. In other words, they continuously contract and expand, shedding some of their mantle of gas and dust. This gas and dust is caught by the star’s rotation and spun into an accretion disc – which might be more properly referred to as a proto-planetary disc. By the time the jets have finished spewing and the material is pulled back to the star by gravity, the proto-star will have cooled enough to have reached main sequence and the pressure may have allowed planetoids to form from the accreted material.
Sunday, February 26 – Today is the birth date of Camille Flammarion. Born in 1842, he became a widely read author of astronomy and originated the idea that we were not alone – the notion of extraterrestrial intelligence. Yet, Flammarion was more than the great grandfather of SETI. In 1877, Flammarion found Charles Messier’s personal notes and catalog in an antiquarian book store. Based on those notes, he was able to identify M102 as Dreyer’s NGC 5866 and associate NGC 4594 with M104. Because of Flammarion’s hard work of scholarship and astronomical observation, two previously obscure references to faint studies in the Messier Catalogue were properly identified.
To locate these two studies, you’ll be waiting until around local midnight. Start at Iota Draconis and head about half a fistwidth in the direction of bright Arcturus to a solitary 5.2 magnitude star. Small, 10th magnitude M102 is about one degree due north toward Polaris. M104 – the “Sombrero Galaxy” – is just a bit more than a fistwidth west of Spica. At magnitude 8.3, it can be easily seen as a small faint glow in binoculars or finderscope. But it requires a telescope and a dark sky to hint at its namesake.
While you’re waiting for them to rise, relax and enjoy the Delta Leonid meteor shower. Entering our atmosphere at speeds of up to 24 kilometers per second, these slow travelers will seem to radiate from a point around the middle of Leo’s “back.” The fall rate is rather slow at 5 per hour, but any meteor trail is a delight to catch!
May all your journeys be at light speed… ~Tammy Plotner. With Jeff Barbour @astro.geekjoy.com