The farthest planet from the Sun that can be observed with the naked eye, the existence of Saturn has been known for thousands of years. And much like all celestial bodies that can be observed with the aid of instruments – i.e. Mercury, Venus, Mars, Jupiter and the Moon – it has played an important role in the mythology and astrological systems of many cultures.
Saturn is one of the four gas giants in our Solar System, also known as the Jovian planets, and the sixth planet from the Sun. It’s ring system, which is it famous for, is also the most observable – consisting of nine continuous main rings and three discontinuous arcs.
Saturn’s Size, Mass and Orbit:
With a polar radius of 54364±10 km and an equatorial radius of 60268±4 km, Saturn has a mean radius of 58232±6 km, which is approximately 9.13 Earth radii. At 5.6846×1026 kg, and a surface area, at 4.27×1010 km2, it is roughly 95.15 as massive as Earth and 83.703 times it’s size. However, since it is a gas giant, it has significantly greater volume – 8.2713×1014 km3, which is equivalent to 763.59 Earths.
The sixth most distant planet, Saturn orbits the Sun at an average distance of 9 AU (1.4 billion km; 869.9 million miles). Due to its slight eccentricity, the perihelion and aphelion distances are 9.022 (1,353.6 million km; 841.3 million mi) and 10.053 AU (1,513,325,783 km; 940.13 million mi), on average respectively.
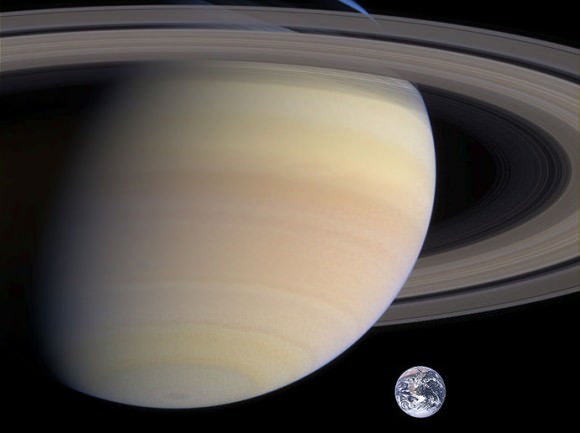
With an average orbital speed of 9.69 km/s, it takes Saturn 10,759 Earth days to complete a single revolution of the Sun. In other words, a single Cronian year is the equivalent of about 29.5 Earth years. However, as with Jupiter, Saturn’s visible features rotate at different rates depending on latitude, and multiple rotation periods have been assigned to various regions.
The latest estimate of Saturn’s rotation as a whole are based on a compilation of various measurements from the Cassini, Voyager and Pioneer probes. Saturn’s rotation causes it to have the shape of an oblate spheroid; flattened at the poles but bulging at the equator.
Saturn’s Composition:
As a gas giant, Saturn is predominantly composed of hydrogen and helium gas. With a mean density of 0.687 g/cm3, Saturn is the only planet in the Solar System that is less dense than water; which means that it lacks a definite surface, but is believed to have a solid core. This is due to the fact that Saturn’s temperature, pressure, and density all rise steadily toward the core.
Standard planetary models suggest that the interior of Saturn is similar to that of Jupiter, having a small rocky core surrounded by hydrogen and helium with trace amounts of various volatiles. This core is similar in composition to the Earth, but more dense due to the presence of metallic hydrogen, which as a result of the extreme pressure.

Saturn has a hot interior, reaching 11,700 °C at its core, and it radiates 2.5 times more energy into space than it receives from the Sun. This is due in part to the Kelvin-Helmholtz mechanism of slow gravitational compression, but may also be attributable to droplets of helium rising from deep in Saturn’s interior out to the lower-density hydrogen. As these droplets rise, the process releases heat by friction and leaves Saturn’s outer layers depleted of helium. These descending droplets may have accumulated into a helium shell surrounding the core.
In 2004, French astronomers Didier Saumon and Tristan Guillot estimated that the core must 9-22 times the mass of Earth, which corresponds to a diameter of about 25,000 km. This is surrounded by a thicker liquid metallic hydrogen layer, followed by a liquid layer of helium-saturated molecular hydrogen that gradually transitions to a gas with increasing altitude. The outermost layer spans 1,000 km and consists of gas.
Saturn’s Atmosphere:
The outer atmosphere of Saturn contains 96.3% molecular hydrogen and 3.25% helium by volume. The gas giant is also known to contain heavier elements, though the proportions of these relative to hydrogen and helium is not known. It is assumed that they would match the primordial abundance from the formation of the Solar System.
Trace amounts of ammonia, acetylene, ethane, propane, phosphine and methane have been also detected in Saturn’s atmosphere. The upper clouds are composed of ammonia crystals, while the lower level clouds appear to consist of either ammonium hydrosulfide (NH4SH) or water. Ultraviolet radiation from the Sun causes methane photolysis in the upper atmosphere, leading to a series of hydrocarbon chemical reactions with the resulting products being carried downward by eddies and diffusion.
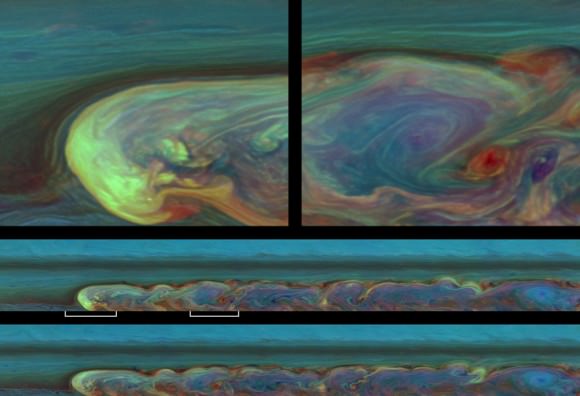
Saturn’s atmosphere exhibits a banded pattern similar to Jupiter’s, but Saturn’s bands are much fainter and wider near the equator. As with Jupiter’s cloud layers, they are divided into the upper and lower layers, which vary in composition based on depth and pressure. In the upper cloud layers, with temperatures in range of 100–160 K and pressures between 0.5–2 bar, the clouds consist of ammonia ice.
Water ice clouds begin at a level where the pressure is about 2.5 bar and extend down to 9.5 bar, where temperatures range from 185–270 K. Intermixed in this layer is a band of ammonium hydrosulfide ice, lying in the pressure range 3–6 bar with temperatures of 290–235 K. Finally, the lower layers, where pressures are between 10–20 bar and temperatures are 270–330 K, contains a region of water droplets with ammonia in an aqueous solution.
On occasion, Saturn’s atmosphere exhibits long-lived ovals, similar to what is commonly observed on Jupiter. Whereas Jupiter has the Great Red Spot, Saturn periodically has what’s known as the Great White Spot (aka. Great White Oval). This unique but short-lived phenomenon occurs once every Saturnian year, roughly every 30 Earth years, around the time of the northern hemisphere’s summer solstice.
These spots can be several thousands of kilometers wide, and have been observed in 1876, 1903, 1933, 1960, and 1990. Since 2010, a large band of white clouds called the Northern Electrostatic Disturbance have been observed enveloping Saturn, which was spotted by the Cassini space probe. If the periodic nature of these storms is maintained, another one will occur in about 2020.
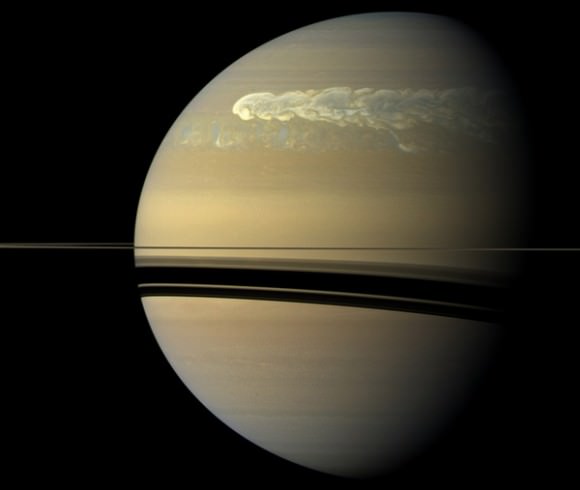
The winds on Saturn are the second fastest among the Solar System’s planets, after Neptune’s. Voyager data indicate peak easterly winds of 500 m/s (1800 km/h). Saturn’s northern and southern poles have also shown evidence of stormy weather. At the north pole, this takes the form of a hexagonal wave pattern, whereas the south shows evidence of a massive jet stream.
The persisting hexagonal wave pattern around the north pole was first noted in the Voyager images. The sides of the hexagon are each about 13,800 km (8,600 mi) long (which is longer than the diameter of the Earth) and the structure rotates with a period of 10h 39m 24s, which is assumed to be equal to the period of rotation of Saturn’s interior.
The south pole vortex, meanwhile, was first observed using the Hubble Space Telescope. These images indicated the presence of a jet stream, but not a hexagonal standing wave. These storms are estimated to be generating winds of 550 km/h, are comparable in size to Earth, and believed to have been going on for billions of years. In 2006, the Cassini space probe observed a hurricane-like storm that had a clearly defined eye. Such storms had not been observed on any planet other than Earth – even on Jupiter.
Saturn’s Moons:
Saturn has at least 150 moons and moonlets, but only 53 of these moons have been given official names. Of these moons, 34 are less than 10 km in diameter and another 14 are between 10 and 50 km in diameter. However, some of its inner and outer moons are rather large, ranging from 250 to over 5000 km.
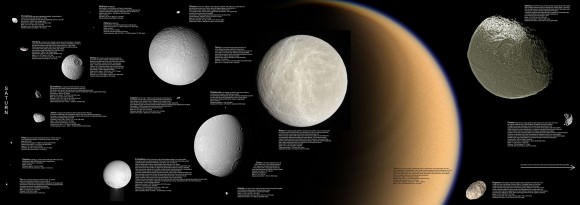
Traditionally, most of Saturn’s moons have been named after the Titans of Greek mythology, and are grouped based on their size, orbits, and proximity to Saturn. The innermost moons and regular moons all have small orbital inclinations and eccentricities and prograde orbits. Meanwhile, the irregular moons in the outermost regions have orbital radii of millions of kilometers, orbital periods lasting several years, and move in retrograde orbits.
The Inner Large Moons, which orbit within the E Ring (see below), includes the larger satellites Mimas, Enceladus, Tethys, and Dione. These moons are all composed primarily of water ice, and are believed to be differentiated into a rocky core and an icy mantle and crust. With a diameter of 396 km and a mass of 0.4×1020 kg, Mimas is the smallest and least massive of these moons. It is ovoid in shape and orbits Saturn at a distance of 185,539 km with an orbital period of 0.9 days.
Enceladus, meanwhile, has a diameter of 504 km, a mass of 1.1×1020 km and is spherical in shape. It orbits Saturn at a distance of 237,948 km and takes 1.4 days to complete a single orbit. Though it is one of the smaller spherical moons, it is the only Cronian moon that is endogenously active – and one of the smallest known bodies in the Solar System that is geologically active. This results in features like the famous “tiger stripes” – a series of continuous, ridged, slightly curved and roughly parallel faults within the moon’s southern polar latitudes.
Large geysers have also been observed in the southern polar region that periodically release plumes of water ice, gas and dust which replenish Saturn’s E ring. These jets are one of several indications that Enceladus has liquid water beneath it’s icy crust, where geothermal processes release enough heat to maintain a warm water ocean closer to its core. With a geometrical albedo of more than 140%, Enceladus is one of the brightest known objects in the Solar System.
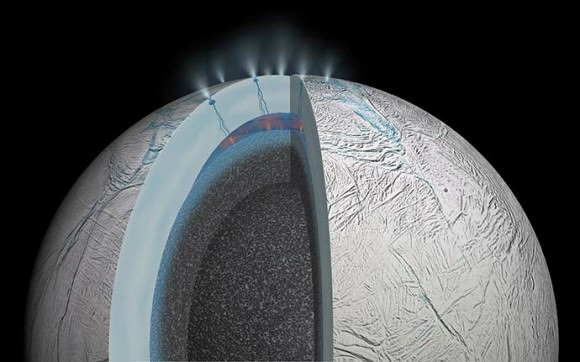
At 1066 km in diameter, Tethys is the second-largest of Saturn’s inner moons and the 16th-largest moon in the Solar System. The majority of its surface is made up of heavily cratered and hilly terrain and a smaller and smoother plains region. Its most prominent features are the large impact crater of Odysseus, which measures 400 km in diameter, and a vast canyon system named Ithaca Chasma – which is concentric with Odysseus and measures 100 km wide, 3 to 5 km deep and 2,000 km long.
With a diameter and mass of 1,123 km and 11×1020 kg, Dione is the largest inner moon of Saturn. The majority of Dione’s surface is heavily cratered old terrain, with craters that measure up to 250 km in diameter. However, the moon is also covered with an extensive network of troughs and lineaments which indicate that in the past it had global tectonic activity.
The Large Outer Moons, which orbit outside of the Saturn’s E Ring, are similar in composition to the Inner Moons – i.e. composed primarily of water ice and rock. Of these, Rhea is the second largest – measuring 1,527 km in diameter and 23 × 1020 kg in mass – and the ninth largest moon of the Solar System. With an orbital radius of 527,108 km, it is the fifth-most distant of the larger moons, and takes 4.5 days to complete an orbit.
Like other Cronian satellites, Rhea has a rather heavily cratered surface, and a few large fractures on its trailing hemisphere. Rhea also has two very large impact basins on its anti-Saturnian hemisphere – the Tirawa crater (similar to Odysseus on Tethys) and an as-yet unnamed crater – that measure 400 and 500 km across, respectively.
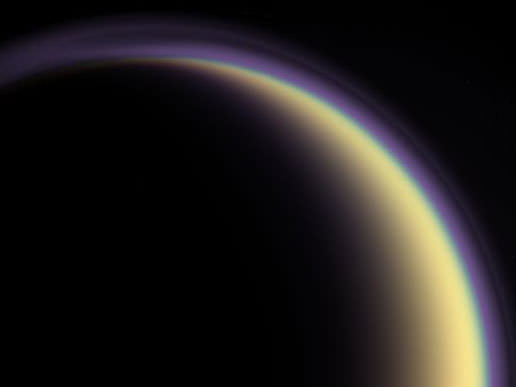
At 5150 km in diameter, and 1,350×1020 kg in mass, Titan is Saturn’s largest moon and comprises more than 96% of the mass in orbit around the planet. Titan is also the only large moon to have its own atmosphere, which is cold, dense, and composed primarily of nitrogen with a small fraction of methane. Scientists have also noted the presence of polycyclic aromatic hydrocarbons in the upper atmosphere, as well as methane ice crystals.
The surface of Titan, which is difficult to observe due to persistent atmospheric haze, shows only a few impact craters, evidence of cryo-volcanoes, and longitudinal dune fields that were apparently shaped by tidal winds. Titan is also the only body in the Solar System beside Earth with bodies of liquid on its surface, in the form of methane–ethane lakes in Titan’s north and south polar regions.
With an orbital distance of 1,221,870 km, it is the second-farthest large moon from Saturn, and completes a single orbit every 16 days. Like Europa and Ganymede, it is believed that Titan has a subsurface ocean made of water mixed with ammonia, which can erupt to the surface of the moon and lead to cryovolcanism.
Hyperion is Titan’s immediate neighbor. At an average diameter of about 270 km, it is smaller and lighter than Mimas. It is also irregularly shaped and quite odd in composition. Essentially, the moon is an ovoid, tan-colored body with an extremely porous surface (which resembles a sponge). The surface of Hyperion is covered with numerous impact craters, most of which are 2 to 10 km in diameter. It also has a highly unpredictable rotation, with no well-defined poles or equator.
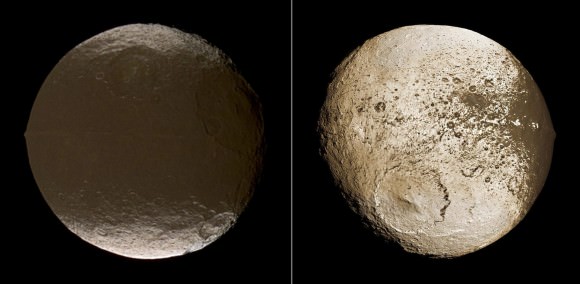
At 1,470 km in diameter and 18×1020 kg in mass, Iapetus is the third-largest of Saturn’s large moons. And at a distance of 3,560,820 km from Saturn, it is the most distant of the large moons, and takes 79 days to complete a single orbit. Due to its unusual color and composition – its leading hemisphere is dark and black whereas its trailing hemisphere is much brighter – it is often called the “yin and yang” of Saturn’s moons.
Beyond these larger moons are Saturn’s Irregular Moons. These satellites are small, have large-radii, are inclined, have mostly retrograde orbits, and are believed to have been acquired by Saturn’s gravity. These moons are made up of three basic groups – the Inuit Group, the Gallic Group, and the Norse Group.
The Inuit Group consists of five irregular moons that are all named from Inuit mythology – Ijiraq, Kiviuq, Paaliaq, Siarnaq, and Tarqeq. All have prograde orbits that range from 11.1 to 17.9 million km, and from 7 to 40 km in diameter. They are all similar in appearance (reddish in hue) and have orbital inclinations of between 45 and 50°.
The Gallic group are a group of four prograde outer moons named for characters in Gallic mythology -Albiorix, Bebhionn, Erriapus, and Tarvos. Here too, the moons are similar in appearance and have orbits that range from 16 to 19 million km. Their inclinations are in the 35°-40° range, their eccentricities around 0.53, and they range in size from 6 to 32 km.
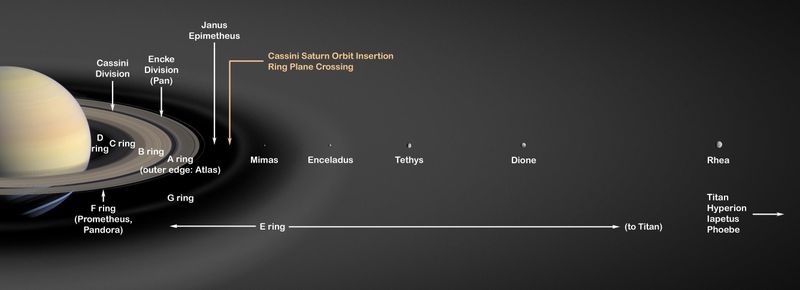
Last, there is the Norse group, which consists of 29 retrograde outer moons that take their names from Norse mythology. These satellites range in size from 6 to 18 km, their distances from 12 and 24 million km, their inclinations between 136° and 175°, and their eccentricities between 0.13 and 0.77. This group is also sometimes referred to as the Phoebe group, due to the presence of a single larger moon in the group – which measures 240 km in diameter. The second largest, Ymir, measures 18 km across.
Within the Inner and Outer Large Moons, there are also those belonging to Alkyonide group. These moons – Methone, Anthe, and Pallene – are named after the Alkyonides of Greek mythology, are located between the orbits of Mimas and Enceladus, and are among the smallest moons around Saturn.
Some of the larger moons even have moons of their own, which are known as Trojan moons. For instance, Tethys has two trojans – Telesto and Calypso, while Dione has Helene and Polydeuces.
Saturn’s Ring System:
Saturn’s rings are believed to be very old, perhaps even dating back to the formation of Saturn itself. There are two main theories as to how these rings formed, each of which have variations. One theory is that the rings were once a moon of Saturn whose orbit decayed until it came close enough to be ripped apart by tidal forces.
In version of this theory, the moon was struck by a large comet or asteroid – possible during the Late Heavy Bombardment – that pushed it beneath the Roche Limit. The second theory is that the rings were never part of a moon, but are instead left over from the original nebular material from which Saturn formed billions of years ago.
The structure is subdivided into seven smaller ring sets, each of which has a division (or gap) between it and its neighbor. The A and B Rings are the densest part of the Cronian ring system and are 14,600 and 25,500 km in diameter, respectively. They extend to a distance of 92,000 – 117,580 km (B Ring) and 122,170 – 136,775 km (A Ring) from Saturn’s center, and are separated by the 4,700 km wide Cassini Division.

The C Ring, which is separated from the B Ring by the 64 km Maxwell Gap, is approximately 17,500 km in width and extends 74,658 – 92,000 from Saturn’s center. Together with the A and B Rings, they comprise the main rings, which are denser and contain larger particles than the “dusty rings”.
These tenuous rings are called “dusty” due to the small particles that make them up. They include the D Ring, a 7,500 km ring that extends inward to Saturn’s cloud tops (66,900 – 74,510 km from Saturn’s center) and is separated from the C Ring by the 150 km Colombo Gap. On the other end of the system, the G and E Rings are located, which are also “dusty” in composition.
The G Ring is 9000 km in width and extends 166,000 – 175,000 km from Saturn’s center. The E Ring, meanwhile, is the largest single ring section, measuring 300,000 km in width and extending 166,000 to 480,000 km from Saturn’s center. It is here where the majority of Saturn’s moons are located (see above).
The narrow F Ring, which sits on the outer edge of the A Ring, is more difficult to categorize. While some parts of it are very dense, it also contains a great deal of dust-size particles. For this reason, estimates on its width range from 30 to 500 km, and it extends roughly 140,180 km from Saturn’s center.
History of Observing Saturn:
Because it is visible to the naked eye in the night sky, human beings have been observing Saturn for thousands of years. In ancient times, it was considered the most distant of five known the planets, and thus was accorded special meaning in various mythologies. The earliest recorded observations come from the Babylonians, where astronomers systematically observed and recorded its movements through the zodiac.

To the ancient Greeks, this outermost planet was named Cronus (Kronos), after the Greek god of agriculture and youngest of the Titans. The Greek scientist Ptolemy made calculations of Saturn’s orbit based on observations of the planet while it was in opposition.The Romans followed in this tradition, identifying it with their equivalent of Cronos (named Saturnus).
In ancient Hebrew, Saturn is called ‘Shabbathai’, whereas in Ottoman Turkish, Urdu and Malay, its name is ‘Zuhal’, which derived is from the original Arabic. In Hindu astrology, there are nine astrological objects known as Navagrahas. Saturn, which is one of them, is known as “Shani”, who judges everyone based on the good and bad deeds performed in life. In ancient China and Japan, the planet was designated as the “earth star” – based on the Five Elements of earth, air, wind, water and fire.
However, the planet was not directly observed until 1610, when Galileo Galilee first discerned the presence of rings. At the time, he mistook them for two moons that were located on either side. It was not until Christiaan Huygens used a telescope with greater magnification that this was corrected. Huygens also discovered Saturn’s moon Titan, and Giovanni Domenico Cassini later discovered the moons of Iapetus, Rhea, Tethys and Dione.
No further discoveries of significance were made again until the 181th and 19th centuries. The first occurred in 1789 when William Herschel discovered the two distant moons of Mimas and Enceladus, and then in 1848 when a British team discovered the irregularly-shaped moon of Hyperion.
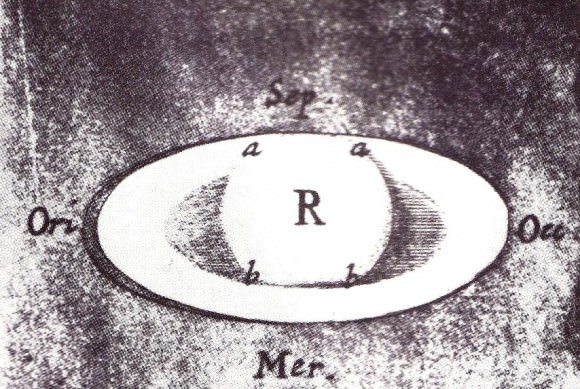
In 1899 William Henry Pickering discovered Phoebe, noting that it had a highly irregular orbit that did not rotate synchronously with Saturn as the larger moons do. This was the first time any satellite had been found to move about a planet in retrograde orbit. And by 1944, research conducted throughout the early 20th century confirmed that Titan has a thick atmosphere – a feature unique among the Solar System’s moons.
Exploration of Saturn:
By the late 20th century, unmanned spacecraft began to conduct flybys of Saturn, gathering information on its composition, atmosphere, ring structure, and moons. The first flyby was conducted by NASA using the Pioneer 11 robotic space probe, which passed Saturn at a distance of 20,000 km in September of 1979.
Images were taken of the planet and a few of its moons, although their resolution was too low to discern surface detail. The spacecraft also studied Saturn’s rings, revealing the thin F Ring and the fact that dark gaps in the rings are bright when facing towards the Sun, meaning that they contain fine light-scattering material. In addition, Pioneer 11 measured the temperature of Titan.
The next flyby took place in November of 1980 when the Voyager 1 space probe passed through the Saturn system. It sent back the first high-resolution images of the planet, its rings and satellites – which included features of various moons that had never before been seen.
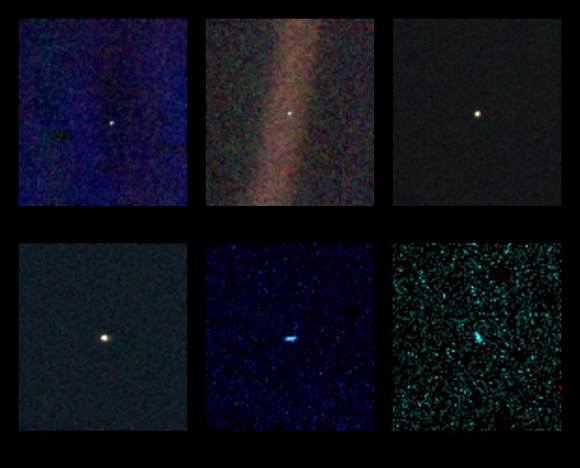
In August 1981, Voyager 2 conducted its flyby and gathered more close-up images of Saturn’s moons, as well as evidence of changes in the atmosphere and the rings. The probes discovered and confirmed several new satellites orbiting near or within the planet’s rings, as well as the small Maxwell Gap and Keeler gap (a 42 km wide gap in the A Ring).
In June of 2004, the Cassini–Huygens space probe entered the Saturn system and conducted a close flyby of Phoebe, sending back high-resolution images and data. By July 1st, 2004, the probe entered orbit around Saturn, and by December, it had completed two flybys of Titan before releasing the Huygens probe. This lander reached the surface and began transmitting data on the atmospheric and surface by by Jan. 14th, 2005. Cassini has since conducted multiple flybys of Titan and other icy satellites.
In 2006, NASA reported that Cassini had found evidence of liquid water reservoirs that erupt in geysers on Saturn’s moon Enceladus. Over 100 geysers have since been identified, which are concentrated around the southern polar region. In May 2011, NASA scientists at an Enceladus Focus Group Conference reported that Enceladus’ interior ocean may be the most likely candidate in the search for extra-terrestrial life.
In addition, Cassini photographs have revealed a previously undiscovered planetary ring, eight new satellites, and evidence of hydrocarbon lakes and seas near Titan’s north pole. The probe was also responsible for sending back high-resolution images of the intense storm activity at Saturn’s northern and southern poles.
Cassini’s primary mission ended in 2008, but the probe’s mission has been extended twice since then – first to September 2010 and again to 2017. In the coming years, NASA hopes to use the probe to study a full period of Saturn’s seasons.

From being a very important part of the astrological systems of many cultures to becoming the subject of ongoing scientific fascination, Saturn continues to occupy a special place in our hearts and minds. Whether it’s Saturn’s fantastically large and beautiful ring system, its many many moons, its tempestuous weather, or its curious composition, this gas giant continues to fascinate and inspire.
In the coming years and decades, additional robotic explorer missions will likely to be sent to investigate Saturn, its rings and its system of moons in greater detail. What we find may constitute some of the most groundbreaking discoveries of all time, and will likely teach us more about the history of our Solar System.
Universe Today has articles on the density of Saturn, the Orbit of Saturn, and Interesting Facts about Saturn.
If you want to learn more about Saturn’s rings and moons, check out Where Did Saturn’s Rings Come From? and How Many Moons Does Saturn Have?
For more information, check out Saturn and all about Saturn, and NASA’s Solar System Exploration page on Saturn.
Astronomy Cast has an episode on the subject – Episode 59: Saturn.