What if it were possible to just suck all the harmful pollutants out of the air so that they wouldn’t be such a nuisance? What if it were also possible to convert these atmospheric pollutants back into fossil fuels, or possibly ecologically-friendly bio fuels? Why, then we would be able to worry far less about smog, respiratory illnesses, and the effects that high concentrations of these gases have on the planet.
This is the basis of Carbon Capture, a relatively new concept where carbon dioxide is captured at point sources – such as factories, natural-gas plants, fuel plants, major cities, or any other place where large concentrations of CO² are known to be found. This CO² can then be stored for future use, converted into biofuels, or simply put back into the Earth so that it doesn’t enter the atmosphere.
Description:
Like many other recent developments, carbon capture is part of a new set of procedures that are collectively known as geoengineering. The purpose of these procedures are to alter the climate to counteract the effects of global warming, generally by targeting one of the chief greenhouse gases. The technology has existed for some time, but it has only been in recent years that it has been proposed as a means of combating climate change as well.
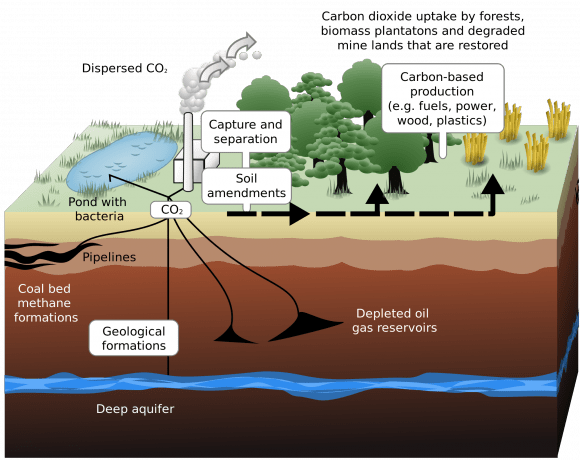
Currently, carbon capture is most often employed in plants that rely on fossil fuel burning to generate electricity. This process is performed in one of three basic ways – post-combustion, pre-combustion and oxy-fuel combustion. Post-combustion involves removing CO2 after the fossil fuel is burned and is converted into a flue gas, which consists of CO2, water vapor, sulfur dioxides and nitrogen oxide.
When the gases travel through a smokestack or chimney, CO² is captured by a “filter” which actually consists of solvents that are used to absorb CO2 and water vapor. This technique is effective in that such filters can be retrofitted to older plants, avoiding the need for a costly power plant overhaul.
Benefits and Challenges:
The results of these processes have so far been encouraging – which boast the possibility of up to 90 % of CO² being removed from emissions (depending on the type of plant and the method used). However, there are concerns that some of these processes add to the overall cost and energy consumption of power plants.
According to 2005 report by the Intergovernmental Panel on Climate Change (IPCC), the additional costs range from 24 to 40% for coal power plants, 11 to 22% for natural gas plants, and 14 to 25% for coal-based gasification combined cycle systems. The additional power consumption also creates more in the way of emissions.
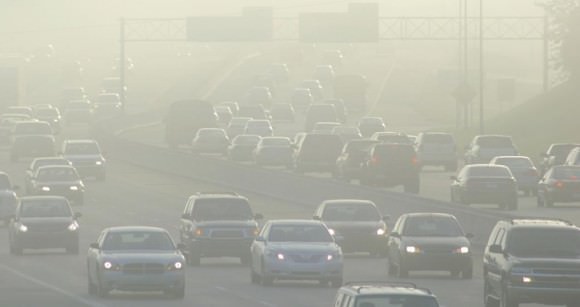
In addition, while CC operations are capable of drastically reducing CO², they can add other pollutants to the air. The amounts of kind of pollutants depend on the technology, and range from ammonia and nitrogen oxides (NO and NO²) to sulfur oxides and disulfur oxides (SO, SO², SO³, S²O, S²O³. etc.). However, researchers are developing new techniques which they hope will reduce both costs and consumption and not generate additional pollutants.
Examples:
A good example of the Carbon Capture process is the Petro Nova project, a coal-fired power plant in Texas. This plant began being upgraded by the US Dept. of Energy (DOE) in 2014 to accommodate the largest post-combustion carbon-capture operation in the world.
Consisting of filters that would capture the emissions, and infrastructure that would place it back in the Earth, the DOE estimates that this operation will be capable of capturing 1.4 million tons of CO2 that previously would have been released into the air.
In the case of pre-combustion, CO² is trapped before the fossil fuel is even burned. Here, coal, oil or natural gas is heated in pure oxygen, resulting in a mix of carbon monoxide and hydrogen. This mix is then treated in a catalytic converter with steam, which then produces more hydrogen and carbon dioxide.
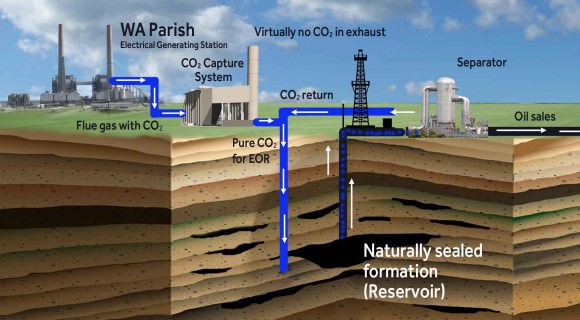
These gases are then fed into flasks where they are treated with amine (which binds with the CO² but not hydrogen); the mixture is then heated, causing the CO² to rise where it can be collected. In the final process (oxy-fuel combustion), fossil fuel is burned in oxygen, resulting in a gas mixture of steam and CO². The steam and carbon dioxide are separated by cooling and compressing the gas stream, and once separated, the CO² is removed.
Other efforts at carbon capture include building urban structures with special facilities to extract CO² from the air. Examples of this include the Torre de Especialidades in Mexico City – a hospital that is surrounded by a 2500 m² facade composed of Prosolve370e. Designed by Berlin-based firm Elegant Embellishments, this specially-shaped facade is able to channel air through its lattices and relies on chemical processes to filter out smog.
China’s Phoenix Towers – a planned-project for a series of towers in Wuhan, China (which will also be the world’s tallest) – is also expected to be equipped with a carbon capture operation. As part of the designers vision of creating a building that is both impressively tall and sustainable, these include special coatings on the outside of the structures that will draw CO² out of the local city air.
Then there’s the idea for “artificial trees“, which was put forward by Professor Klaus Lackner of the Department of Earth and Environmental Engineering at Columbia University. Consisting of plastic fronds that are coated with a resin that contains sodium carbonation – which when combined with carbon dioxide creates sodium bicarbonate (aka. baking soda) – these “trees” consume CO² in much the same way real trees do.
A cost-effective version of the same technology used to scrub CO² from air in submarines and space shuttles, the fronds are then cleaned using water which, when combined with the sodium bicarbonate, yields a solution that can easily be converted into biofuel.
In all cases, the process of Carbon Capture comes down to finding ways to remove harmful pollutants from the air to reduce humanity’s footprint. Storage and reuse also enter into the equation in the hopes of giving researchers more time to develop alternative energy sources.
We have written many interesting articles about carbon capture here at Universe Today. Here’s What is Carbon Dioxide?, What Causes Air Pollution?, What if we Burn Everything?, Global Warming Watch: How Carbon Dioxide Bleeds Across The Earth, and World Needs to Aim for Near-Zero Carbon Emissions.
For more information on how Carbon Capture works, be sure to check out this video from the Carbon Capture and Storage Organization:
If you’d like more info on Earth, check out NASA’s Solar System Exploration Guide on Earth. And here’s a link to NASA’s Earth Observatory.
We’ve also have Astronomy Cast episodes all about planet Earth and Climate Change. Listen here, Episode 51: Earth, Episode 308: Climate Change.
Sources: