[/caption]
It has been reported that a recent scientific paper delivers the conclusion that our universe resides inside a black hole in another universe. In fact, this isn’t really what the paper concluded – although what the paper did conclude is still a little out of left field.
The Einstein-Cartan-Kibble-Sciama (ECKS) theory of gravity – claimed as an alternative to general relativity theory, although still based on Einstein field equations – seeks to take greater account of the effect of the spin of massive particles. Essentially, while general relativity has it that matter determines how spacetime curves, ECKS also tries to capture the torsion of spacetime, which is a more dynamic idea of curvature – where you have to think in terms of twisting and contortion, rather than just curvature.
Mind you, general relativity is also able to deal with dynamic curvature. ECKS proponents claim that where ECKS departs from general relativity is in situations with very high matter density – such as inside black holes. General relativity suggests that a singularity (with infinite density and zero volume) forms beyond a black hole’s event horizon. This is not a very satisfying result since the contents of black holes do seem to occupy volume – more massive ones have larger diameters than less massive ones – so general relativity may just not be up to the task of dealing with black hole physics.
ECKS theory attempts to step around the singularity problem by proposing that an extreme torsion of spacetime, resulting from the spin of massive particles compressed within a black hole, prevents a singularity from forming. Instead the intense compression increases the intrinsic angular momentum of the matter within (i.e. the spinning skater draws arms in analogy) until a point is reached where spacetime becomes as twisted, or as wound up, as it can get. From that point the tension must be released through an expansion (i.e. an unwinding) of spacetime in a whole new tangential direction – and voila you get a new baby universe.
But the new baby universe can’t be born and expand in the black hole. Remember this is general relativity. From any frame of reference outside the black hole, the events just described cannot sequentially happen. Clocks seem to slow to a standstill as they approach a black hole’s event horizon. It makes no sense for an external observer to imagine that a sequence of events is taking place over time inside a black hole.
Instead, it is proposed that the birth and expansion of new baby universe proceeds along a separate branch of spacetime with the black hole acting as an Einstein-Rosen bridge (i.e. a wormhole).
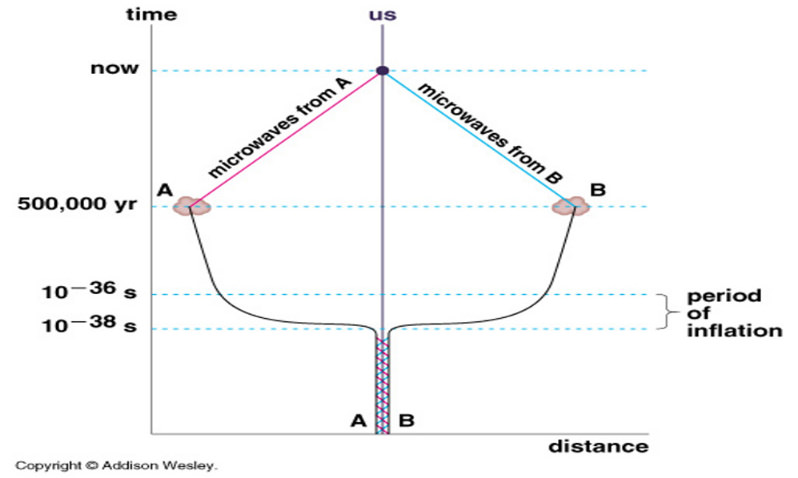
If correct, it’s a turtles on turtles solution and we are left to ponder the mystery of the first primeval universe which first formed the black holes from which all subsequent universes originate.
Something the ECKS hypothesis does manage to do is to provide an explanation for cosmic inflation. Matter and energy crunched within a black hole should achieve a state of isotropy and homogeneity (i.e. no wrinkles) – and when it expands into a new universe through a hypothetical wormhole, this is driven by the unwinding of the spacetime torsion that was built up within the black hole. So you have an explanation for why a universe expands – and why it is so isotropic and homogenous.
Despite there not being the slightest bit of evidence to support it, this does rank as an interesting idea.
Further reading: Poplawski, N.J. (2010) Cosmology with torsion – an alternative to cosmic inflation.