[/caption]
The search for extraterrestrial life outside our Solar System is currently focused on extrasolar planets within the ‘habitable zones’ of exoplanetary systems around stars similar to the Sun. Finding Earth-like planets around other stars is the primary goal of NASA’s Kepler Mission.
The habitable zone (HZ) around a star is defined as the range of distances over which liquid water could exist on the surface of a terrestrial planet, given a dense enough atmosphere. Terrestrial planets are generally defined as rocky and similar to Earth in size and mass. A visualization of the habitable zones around stars of different diameters and brightness and temperature is shown here. The red region is too hot, the blue region is too cold, but the green region is just right for liquid water. Because it can be described this way, the HZ is also referred to as the “Goldilocks Zone”.
Normally, we think of planets around other stars as being similar to our solar system, where a retinue of planets orbits a single star. Although theoretically possible, scientists debated whether or not planets would ever be found around pairs of stars or multiple star systems. Then, in September, 2011, researchers at NASA’s Kepler mission announced the discovery of Kepler-16b, a cold, gaseous, Saturn-sized planet that orbits a pair of stars, like Star Wars’ fictional Tatooine.
This week I had the chance to interview one of the young guns studying exoplanets, Billy Quarles. Monday, Billy and his co-authors, professor Zdzislaw Musielak and associate professor Manfred Cuntz, presented their findings on the possibility of Earth-like planets inside the habitable zones of Kepler 16 and other circumbinary star systems, at the AAS meeting in Austin, Texas.
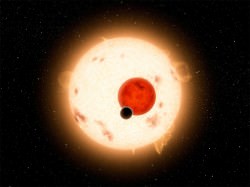
“To define the habitable zone we calculate the amount of flux that is incident on an object at a given distance,” Billy explained. “We also took into account that different planets with different atmospheres will retain heat differently. A planet with a really weak greenhouse effect can be closer in to the stars. For a planet with a much stronger greenhouse effect, the habitable zone will be further out.”
“In our particular study, we have a planet orbiting two stars. One of the stars is much brighter than the other. So much brighter, that we ignored the flux coming from the smaller fainter companion star altogether. So our definition of the habitable zone in this case is a conservative estimate.”
Quarles and his colleagues performed extensive numerical studies on the long-term stability of planetary orbits within the Kepler 16 HZ. “The stability of the planetary orbit depends on the distance from the binary stars,” said Quarles. “The further out the more stable they tend to be, because there is less perturbation from the secondary star.”
For the Kepler 16 system, planetary orbits around the primary star are only stable out to 0.0675 AU (astronomical units). “That is well inside the inner limit of habitability, where the runaway greenhouse effect takes over,” Billy explained. This all but rules out the possibility of habitable planets in close orbit around the primary star of the pair. What they found was that orbits in the Goldilocks Zone farther out, around the pair of Kepler 16’s low-mass stars, are stable on time scales of a million years or more, providing the possibility that life could evolve on a planet within that HZ.
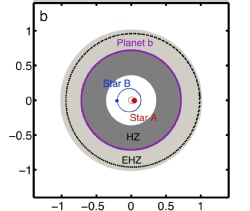
Kepler 16b’s roughly circular orbit, about 65 million miles from the stars, is on the outer edge of this habitable zone. Being a gas giant, 16b is not a habitable terrestrial planet. However, an Earth-like moon, a Goldilocks Moon, in orbit around this planet could sustain life if it were massive enough to retain an Earth-like atmosphere. “We determined that a habitable exomoon is possible in orbit around Kepler-16b,” Quarles said.
I asked Quarles how stellar evolution impacts these Goldilocks Zones. He told me, “There are a number of things to consider over the lifetime of a system. One of them is how the star evolves over time. In most cases the habitable zone starts out close and then slowly drifts out.”
During a star’s main sequence lifetime, nuclear burning of hydrogen builds up helium in its core, causing an increase in pressure and temperature. This occurs more rapidly in stars that are more massive and lower in metallicity. These changes affect the outer regions of the star, which results in a steady increase in luminosity and effective temperature. The star becomes more luminous, causing the HZ to move outwards. This movement could result in a planet within the HZ at the beginning of a star’s main sequence lifetime, to become too hot, and eventually, uninhabitable. Similarly, an inhospitable planet originally outside the HZ, may thaw out and enable life to commence.
“For our study, we ignored the stellar evolution part,” said lead author, Quarles. “We ran our models for a million years to see where the habitable zone was for that part of the star’s life cycle.”
Being at the right distance from its star is only one of the necessary conditions required for a planet to be habitable. Habitable conditions on a planet require various geophysical and geochemical conditions. Many factors can prevent, or impede, habitability. For example, the planet may lack water, gravity may be too weak to retain a dense atmosphere, the rate of large impacts may be too high, or the minimum ingredients necessary for life (still up for debate) may not be there.
One thing is clear. Even with all the requirements for life as we know it, there appear to be plenty of planets around other stars, and very likely, Goldilocks Moons around planets, orbiting within the habitable zones of stars in our galaxy, that detecting the signature of life in the atmosphere of a planet or moon around another Sun seems like only a matter of time now.