Jupiter was appropriately named by the Romans, who chose to name it after the king of the gods. In addition to being the largest planet in our Solar System – with two and a half times the mass of all the other planets combined – it also has the most moons of any Solar planet. So far, 67 natural satellites have been discovered around the gas giant, and more could be on the way.
The moons of Jupiter are so numerous and so diverse that they are broken down into several groups. First, there are the largest moons known as the Galileans, or Main Group. Together with the smaller Inner Group, they make up Jupiter’s Regular Satellites. Beyond them, there are the many Irregular Satellites that circle the planet, along with its debris rings. Here’s what we know about them…
Discovery and Naming:
Using a telescope of his own design, which allowed for 20 x normal magnification, Galileo Galilei was able to make the first observations of celestial bodies that were not visible to the naked eye. In 1610, he made the first recorded discovery of moons orbiting Jupiter, which later came to be known as the Galilean Moons.
At the time, he observed only three objects, which he believed to be fixed stars. However, between January and March of 1610, he continued to observe them, and noted a fourth body as well. In time, he realized that these four bodies did not behave like fixed stars, and were in fact objects that orbited Jupiter.
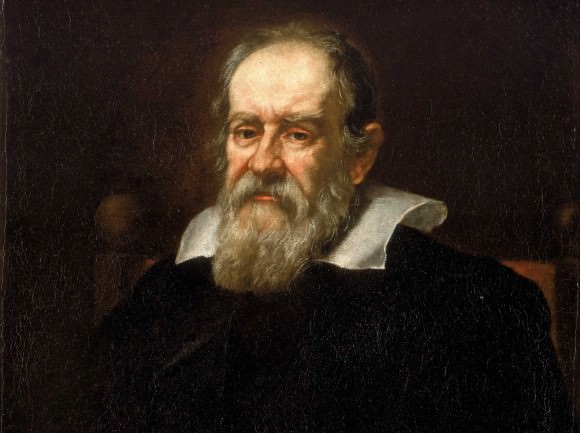
These discoveries proved the importance of using the telescope to view celestial objects that had previously remained unseen. More importantly, by showing that planets other than Earth had their own system of satellites, Galileo dealt a significant blow to the Ptolemaic model of the universe, which was still widely accepted.
Seeking the patronage of the Grand Duke of Tuscany, Cosimo de Medici, Galileo initially sought permission to name the moons the “Cosmica Sidera” (or Cosimo’s Stars). At Cosimo’s suggestion, Galileo changed the name to Medicea Sidera (“the Medician stars”), honouring the Medici family. The discovery was announced in the Sidereus Nuncius (“Starry Messenger”), which was published in Venice in March 1610.
However, German astronomer Simon Marius had independently discovered these moons at the same time as Galileo. At the behest of Johannes Kepler, he named the moons after the lovers of Zues (the Greek equivalent of Jupiter). In his treatise titled Mundus Jovialis (“The World of Jupiter”, published in 1614) he named them Io, Europa, Ganymede, and Callisto.
Galileo steadfastly refused to use Marius’ names and instead invented the numbering scheme that is still used today, alongside proper moon names. In accordance with this scheme, moons are assigned numbers based on their proximity to their parent planet and increase with distance. Hence, the moons of Io, Europa, Ganymede and Callisto were designated as Jupiter I, II, III, and IV, respectively.
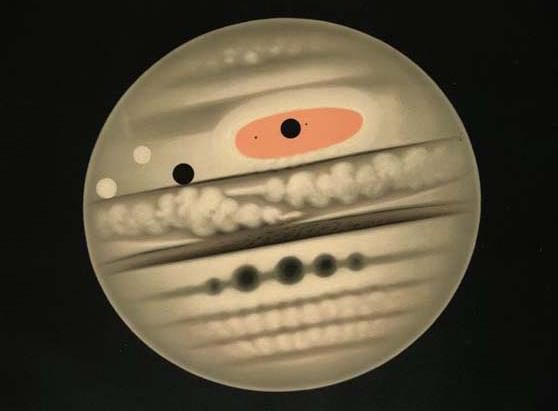
After Galileo made the first recorded discovery of the Main Group, no additional satellites were discovered for almost three centuries – not until E. E. Barnard observed Amalthea in 1892. In fact, it was not until the 20th century, and with the aid of telescopic photography and other refinements, that most of the Jovian satellites began to be discovered.
Himalia was discovered in 1904, Elara in 1905, Pasiphaë in 1908, Sinope in 1914, Lysithea and Carme in 1938, Ananke in 1951, and Leda in 1974. By the time Voyager space probes reached Jupiter around 1979, 13 moons had been discovered, while Voyager herself discovered an additional three – Metis, Adrastea, and Thebe.
Between October 1999 and February 2003, researchers using sensitive ground-based detectors found and later named another 34 moons, most of which were discovered by a team led by Scott S. Sheppard and David C. Jewitt. Since 2003, 16 additional moons have been discovered but not yet named, bringing the total number of known moons of Jupiter to 67.
Though the Galilean moons were named shortly after their discovery in 1610, the names of Io, Europa, Ganymede and Callisto fell out of favor until the 20th century. Amalthea (aka. Jupiter V) was not so named until an unofficial convention took place in 1892, a name that was first used by the French astronomer Camille Flammarion.
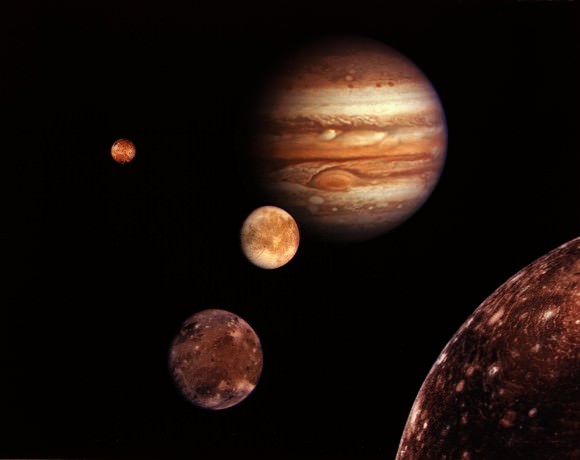
The other moons, in the majority of astronomical literature, were simply labeled by their Roman numeral (i.e. Jupiter IX) until the 1970s. This began in 1975 when the International Astronomical Union’s (IAU) Task Group for Outer Solar System Nomenclature granted names to satellites V–XIII, thus creating a formal naming process for any future satellites discovered. The practice was to name newly discovered moons of Jupiter after lovers and favorites of the god Jupiter (Zeus); and since 2004, also after their descendants.
Regular Satellites:
Jupiter’s Regular Satellites are so named because they have prograde orbits – i.e. they orbit in the same direction as the rotation of their planet. These orbits are also nearly circular and have a low inclination, meaning they orbit close to Jupiter’s equator. Of these, the Galilean Moons (aka. the Main Group) are the largest and the most well known.
These are Jupiter’s largest moons, not to mention the Solar System’s fourth, sixth, first and third largest satellites, respectively. They contain almost 99.999% of the total mass in orbit around Jupiter, and orbit between 400,000 and 2,000,000 km from the planet. They are also among the most massive objects in the Solar System with the exception of the Sun and the eight planets, with radii larger than any of the dwarf planets.
They include Io, Europa, Ganymede, and Callisto, and were all discovered by Galileo Galilei and named in his honor. The names of the moons, which are derived from the lovers of Zeus in Greek mythology, were prescribed by Simon Marius soon after Galileo discovered them in 1610. Of these, the innermost is Io, which is named after a priestess of Hera who became Zeus’ lover.
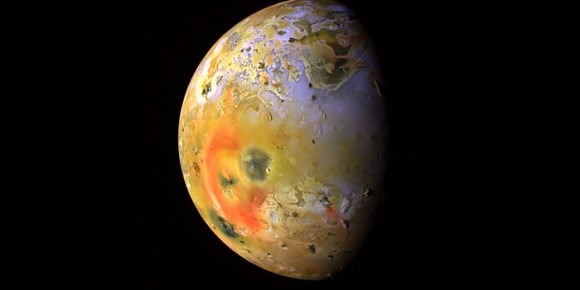
With a diameter of 3,642 kilometers, it is the fourth-largest moon in the Solar System. With over 400 active volcanoes, it is also the most geologically active object in the Solar System. Its surface is dotted with over 100 mountains, some of which are taller than Earth’s Mount Everest.
Unlike most satellites in the outer Solar System (which are covered with ice), Io is mainly composed of silicate rock surrounding a molten iron or iron sulfide core. Io has an extremely thin atmosphere made up mostly of sulfur dioxide (SO2).
The second innermost Galilean moon is Europa, which takes its name from the mythical Phoenician noblewoman who was courted by Zeus and became the queen of Crete. At 3121.6 kilometers in diameter, it is the smallest of the Galileans, and slightly smaller than the Moon.
Europa’s surface consists of a layer of water surrounding the mantle which is thought to be 100 kilometers thick. The uppermost section is solid ice while the bottom is believed to be liquid water, which is made warm due to heat energy and tidal flexing. If true, then it is possible that extraterrestrial life could exist within this subsurface ocean, perhaps near a series of deep-ocean hydrothermal vents.
The surface of Europa is also one of the smoothest in the Solar System, a fact which supports the idea of liquid water existing beneath the surface. The lack of craters on the surface is attributed to the surface being young and tectonically active. Europa is primarily made of silicate rock and likely has an iron core, and a tenuous atmosphere composed primarily of oxygen.
Next up is Ganymede. At 5262.4 kilometers in diameter, Ganymede is the largest moon in the Solar System. While it is larger than the planet Mercury, the fact that it is an icy world means that it has only half of Mercury’s mass. It is also the only satellite in the Solar System known to possess a magnetosphere, likely created through convection within the liquid iron core.
Ganymede is composed primarily of silicate rock and water ice, and a salt-water ocean is believed to exist nearly 200 km below Ganymede’s surface – though Europa remains the most likely candidate for this. Ganymede has a high number of craters, most of which are now covered in ice, and boasts a thin oxygen atmosphere that includes O, O2, and possibly O3 (ozone), and some atomic hydrogen.
Callisto is the fourth and farthest Galilean moon. At 4820.6 kilometers in diameter, it is also the second largest of the Galileans and third largest moon in the Solar System. Callisto is named after the daughter of the Arkadian King, Lykaon, and a hunting companion of the goddess Artemis.
Composed of approximately equal amounts of rock and ices, it is the least dense of the Galileans, and investigations have revealed that Callisto may also have an interior ocean at depths greater than 100 kilometers from the surface.
Callisto is also one of the most heavily cratered satellites in the Solar System – the greatest of which is the 3000 km wide basin known as Valhalla. It is surrounded by an extremely thin atmosphere composed of carbon dioxide and probably molecular oxygen. Callisto has long been considered the most suitable place for a human base for future exploration of the Jupiter system since it is furthest from the intense radiation of Jupiter.
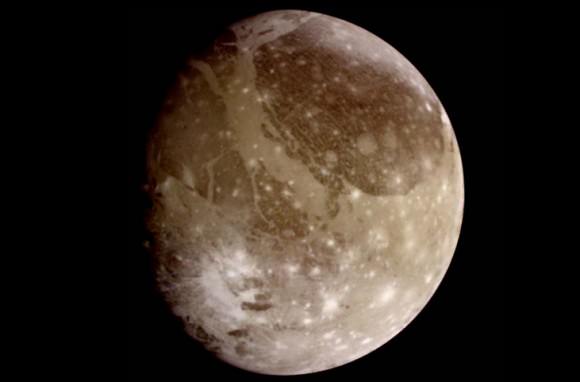
The Inner Group (or Amalthea group) are four small moons that have diameters of less than 200 km, orbit at radii less than 200,000 km, and have orbital inclinations of less than half a degree. This groups includes the moons of Metis, Adrastea, Amalthea, and Thebe.
Along with a number of as-yet-unseen inner moonlets, these moons replenish and maintain Jupiter’s faint ring system – Metis and Adrastea helping Jupiter’s main ring, while Amalthea and Thebe maintain their own faint outer rings.
Metis is the closest moon to Jupiter at a distance of 128,000 km. It is roughly 40 km in diameter, tidally-locked, and highly-asymmetrical in shape (with one of the diameters being almost twice as large as the smallest one). It was not discovered until the 1979 flyby of Jupiter by the Voyager 1 space probe. It was named in 1983 after the first wife of Zeus.
The second closest moon is Adrastea, which is about 129,000 km from Jupiter and 20 km in diameter. Also known as Jupiter XV, Amalthea is the second by distance, and the smallest of the four inner moons of Jupiter. It was discovered in 1979 when the Voyager 2 probe photographed it during a flyby.

Amalthea, also known as Jupiter V, is the third moon of Jupiter in order of distance from the planet. It was discovered on September 9, 1892, by Edward Emerson Barnard and named after a nymph in Greek mythology. It is thought to consist of porous water ice with unknown amounts of other materials. Its surface features include large craters and ridges.
Thebe (aka. Jupiter XIV) is the fourth and final inner moon of Jupiter. It is irregularly shaped and reddish in colour, and is thought like Amalthea to consist of porous water ice with unknown amounts of other materials. Its surface features also include large craters and high mountains – some of which are comparable to the size of the moon itself.
Irregular Satellites:
The Irregular Satellites are those that are substantially smaller and have more distant and eccentric orbits than the Regular Satellites. These moons are broken down into families that have similarities in orbit and composition. It is believed that these were at least partially formed as a result of collisions, most likely by asteroids that were captured by Jupiter’s gravitational field.
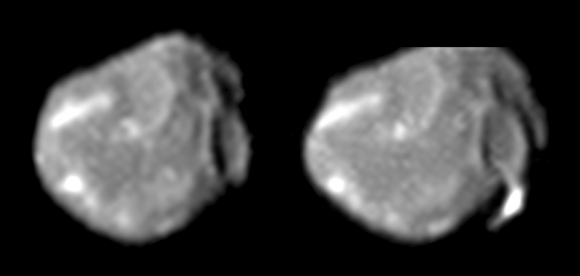
Those that are grouped into families are all named after their largest member. For example, the Himalia group is named after Himalia – a satellite with a mean radius of 85 km, making it the fifth largest moon orbiting Jupiter. It is believed that Himalia was once an asteroid that was captured by Jupiter’s gravity, which then experienced a impact that formed the moons of Leda, Lysithea, and Elara. These moons all have prograde orbits, meaning they orbit in the same direction as Jupiter’s rotation.
The Carme group takes its name from the Moon of the same name. With a mean radius of 23 km, Carme is the largest member of a family of Jovian satellites which have similar orbits and appearance (uniformly red), and are therefore thought to have a common origin. The satellites in this family all have retrograde orbits, meaning they orbit Jupiter in the opposite direction of its rotation.
The Ananke group is named after its largest satellite, which has a mean radius of 14 km. It is believed that Ananke was also an asteroid that was captured by Jupiter’s gravity and then suffered a collision which broke off a number of pieces. Those pieces became the other 15 moons in the Ananke group, all of which have retrograde orbits and appear gray in color.
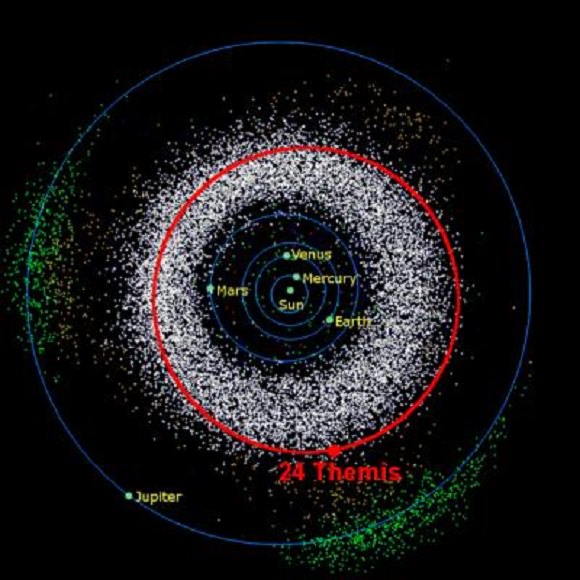
The Pasiphae group is a very diverse group which ranges in color from red to grey – signifying the possibility of it being the result of multiple collisions. Named after Paisphae, which has a mean radius of 30 km, these satellites are retrograde, and are also believed to be the result of an asteroid that was captured by Jupiter and fragmented due to a series of collisions.
There are also several irregular satellites that are not part of any particular family. These include Themisto and Carpo, the innermost and outermost irregular moons, both of which have prograde orbits. S/2003 J 12 and S/2011 J 1 are the innermost of the retrograde moons, while S/2003 J 2 is the outermost moon of Jupiter.
Structure and Composition:
As a rule, the mean density of Jupiter’s moons decrease with their distance from the planet. Callisto, the least dense of the four, has an intermediate density between ice and rock, whereas Io has a density that indicates its made of rock and iron. Callisto’s surface also has a heavily cratered ice surface, and the way it rotates indicates that its density is equally distributed.
This suggests that Callisto has no rocky or metallic core, but consists of a homogeneous mix of ice and rock. The rotation of the three inner moons, in contrast, indicates differentiation between a core of denser matter (such as silicates, rock and metals) and a mantle of lighter material (water ice).
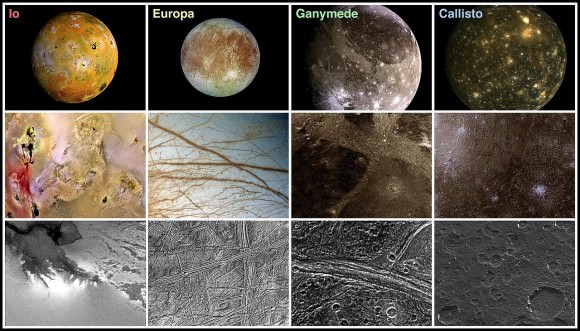
The distance from Jupiter also accords with significant alterations in the surface structure of its moons. Ganymede reveals past tectonic movement of the ice surface, which would mean that the subsurface layers underwent partial melting at once time. Europa reveals more dynamic and recent movement of this nature, suggesting a thinner ice crust. Finally, Io, the innermost moon, has a sulfur surface, active volcanism, and no sign of ice.
All this evidence suggests that the nearer a moon is to Jupiter, the hotter its interior – with models suggesting that the level of tidal heating is in inverse proportion to the square of their distance from the planet. It is believed that all of Jupiter’s moons may have once had an internal composition similar to that of modern-day Callisto, while the rest changed over time as a result of tidal heating caused by Jupiter’s gravitational field.
What this means is that for all of Jupiter’s moons, except Callisto, their interior ice melted, allowing rock and iron to sink to the interior and water to cover the surface. In Ganymede, a thick and solid ice crust then formed while in warmer Europa, a thinner more easily broken crust formed. On Io, the closest planet to Jupiter, the heating was so extreme that all the rock melted and the water boiled out into space.
Jupiter, a gas giant of immense proportions, was appropriately named after the king of the Roman pantheon. It is only befitting that such a planet has many, many moons orbiting it. Given the discovery process, and how long it has taken us, it would not be surprising if there are more satellites around Jupiter just waiting to be discovered. Sixty-seven and counting!
Universe Today has articles on Jupiter’s largest moon and Jupiter moons.
You should also check out Jupiter’s moons and rings and Jupiter’s largest moons.
For more information, try Jupiter’s moons and Jupiter.
Astronomy Cast also has an episode on Jupiter’s moons.