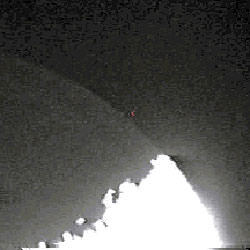
Image credit: Derek Breit. Click to enlarge.
Findings of this nature are one of the many reasons why International Occultation Timing Association (IOTA) members pursue their craft. One of the notable and historic discoveries on a standard star by occultation means happened in 1819 when Antares’ companion star was observed. However, the name of the astronomy game is confirmation – and also filming and timing the northern limit event at differing locations were Walt Morgan and Ed Morana.
Contacting IOTA’s Dr. David Dunham, Breit forwarded his findings, contacted team members and started seeking an answer for two unusual seconds of video. According to Dunham’s response, “Almost 2 seconds with a distance of much more than a km; it’s unlikely that the Moon would be that smooth, it would have to be within about 5m or less for the brightness to remain faint and constant at that level so long. Especially since this apparently occurred at nearly every event, a faint, close companion, only 0.01″ to 0.02″ north of the primary, seems likely.”
And Morgan clarifies, “The disappearances and reappearances by upsilon Geminorum as it passed lunar peaks were usually slow transitions, that is, the star appeared to fade (or brighten) over a matter of several video frames. That was not considered unusual because of the fairly large angular diameter of the star. However, in some instances the magnitude 4.1 star did not seem to completely disappear on Breit’s record: a very faint point of light remained visible right at the lunar limb.”
But confirmation of such importance to the scientific community doesn’t stop there. Breit’s findings went out to all IOTA observers and the critical timing information provided them with the clues they needed. Also recording the event was Dr. Richard Nolthenius, whose answer was, “Derek’s right! I’ve just reduced my upsilon Gem graze video recording from last Friday. I used a PC164c on an 8″ f/10 operating at f/6.3, recorded on my Canon ZR45mc. And the conclusion is…. Derek’s camcorder is not going crazy! I fully confirm his observations and conclusions – this star is a very close double star.”
As they continue to work through the geometry and astrometric angles, Dr. Nolthenius offers the following information from his own recordings: “The second and 3rd D’s look especially like there is an 11th magnitude companion, and the final D most dramatic of all, with the initial fade happening in just 3 frames, followed by a definite but very faint 11th magnitude star left over for fully 1 second before finally disappearing.”
Although it might seem that in a sky filled with innumerable double stars that a revelation of this type would be of little significance, IOTA member – Dr. Michael Richmond – knew better: “I did a little searching to see if there was any other indication that upsilon Geminorum might be double. The Hipparcos observations indicate that it is slightly variable, with an amplitude of about 0.08 mag, but there is no indication of a period. The Astrophysics Data Service has a number of references which mention upsilon Geminorum. This star has been chosen to be a calibrator for optical interferometers; that is, people have decided that it’s a good star to use as a reference when doing high angular resolution measurements. There are two recent papers which list measurements of its angular size: Borde et al. (A&A 393, 183, 2002), which finds an angular diameter of 5.00 +/- 0.051 mas, and Richichi and Percheron (A&A 386, 492, 2002), which lists angular diameter of 5.23 +/- 0.31 mas. Given the Hipparcos parallax of 13.57 mas, this means that the star’s diameter is roughly 0.37 AU. The main star has spectral type listed as late K or early M giant, with V-band mag 4.08 and K-band mag 0.24. If this is a double star, with a companion of roughly mag 11, then it would be important to let other astronomers know: it would no longer be a really good calibration star.”
But, Dr. Richmond did not let his findings rest there and he continued to look for more precise information. Says Richmond, “I found that both of the catalogue entries were NOT based on direct measurements of angular size; instead, they were simply estimates, based on the observed brightness and the shape of the spectrum. In other words, they were basically fits to a blackbody with a given temperature. I was surprised to find such indirect evidence appearing in catalogues of angular size, for use as a calibrator for interferometers.”
Recognizing the importance of such a finding as opposed to known data definitely changes the way we perceive information. Astronomy is a continually upgrading science as Dr. Nolthenius notes: “For some 9th magnitude star, finding yet another double is one thing, but for such a bright star, being a standard for certain measurements should be checked, as you did. The star is apparently in that fall-through-the-cracks area of parameter space: a wide enough double to not make for noticeable periodicity in the radial velocity on a time scale of a few years – the period is likely in the 100+ year range, (although this is something I will calculate later) and yet impossibly difficult as a visual binary without using interferometry or lunar occultations.”
Of course, there is far more to this picture than just the discovery of undisclosed double star. By recording, timing, and observing both grazing and occultation events, IOTA is able to help determine proper movement, orbit and lunar limb features as well. As Dr. Nolthenius explains, “The absolute UT’s of the events will help in assessing the slope of the moon at the event points. However, the most convincing case for duplicity will be identifying significant periods of time of constant brightness at the very faint levels.” The diffraction of large stars aids astronomers in making more accurate calculations, “Perhaps there is a secondary that is of order 1 radius or less above the surface of upsilon Geminorum.” hypothesizes Nolthenius, “If such extended periods of very faint levels might be consistent with limb darkening which is very extended. As a K giant, I would not expect the limb darkening to be so extreme – normally limb darkening is more extreme the cooler the star, and late K is not all that cool.”
More confirmation was needed and the findings were sent to Dr. Mitsuru Soma of the National Astronomical Observatory of Japan. Says Soma, “From the comparison of your faint flash mentioned above and the short duration (0.7s) from R to D of the primary of Walter Morgan the companion’s separation from the primary is estimated to be about 0.04 arcsec, and this is consistent with the duration of your gradual R’s at 4:39:07 and at 4:40:21 (UT). The spectral type of ups Gem is K5III which is the same as Aldebaran according to the Hipparcos catalogue, so I assume that the actual radius of ups Gem is almost the same as Aldebaran. The angular radius of Aldebaran was estimated to be about 0.010 arcsec from lunar occultations.”
But confirmation means being very sure that there is no chance of this being a diffraction effect. As Dr. Soma explains, “The distance to ups Gem is 3.6 times the distance to Aldebaran (ups Gem’s parallax is 0.014 arcsec and Aldebaran’s parallax is 0.050 arcsec) so the angular radius of ups Gem should be about 0.003 arcsec, which is small so that I think the error arisen from the assumption that the star is a point source is almost negligible when we estimate the diffraction effects. Referring to this fact I think 0.04 arcsec I mentioned above is too large to be attributed to the diffraction effects.”
Confirmation continues on a deeper level when Dr. Michael Richmond plots the photometry of all three tapes of the Upsilon Geminorum event: “The thing I find very interesting and encouraging is that I see an asymmetry in these light curves.” says Richmond, “If this is true, then I think we can make a good case that there may be a faint companion to the primary star. The companion must be “ahead” of the primary, so that the moving limb of the moon first blocks (or reveals) the companion, before it blocks (or reveals) the primary.”
Dr. Mitusuru Soma also continued with his analysis and presented the papers at the Journees 2005 meeting in Warsaw on 2005 September 19-21. Based on available information says, “My conclusion about the position of the secondary of upsilon Geminorum relative to the primary is 0″.04 +/- 0″.01 in separation and 70deg +/- 20deg in PA.” Although these findings are preliminary, Soma will continue to review the data and clarify the results of all accumulative information.
Seeing double? The answer is quite probable. In the mean time IOTA members will continue to review of the data and further research the duplicity of upsilon Geminorum. There’s a whole big wide sky out there, and each time an observation of this type is made it adds more to our understanding. While speckle interferometry is cutting edge of double star detection – the occultation method can reveal far more. Contributions from dedicated members are what makes the International Occultation and Timing Association play an important role in today’s astronomy.
Says Breit, “It was a pretty darn good feeling when Dr Nolthenius wrote “Derek’s RIGHT!” When four PhD’s say I have found something special doing a hobby I taught myself from the age of six, that’s pretty good. Something to tell the grandkids… But my real thought was that I finally have a great video to show others and hopefully get them interested in observing these very dynamic and temporal events!” So what are the chances of IOTA members Derek Breit, Walt Morgan, Ed Morana and Michael Richmond making a contribution to the scientific community?
I’d say double.
Written by Tammy Plotner.