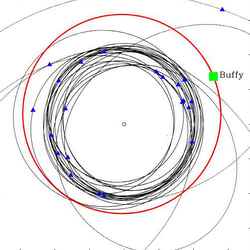
A view of Buffy’s and other Kuiper belt object orbits. Image credit: CFHT Click to enlarge
A team of astronomers working in Canada, France and the United States have discovered an unusual small body orbiting the Sun beyond Neptune, in the region astronomers call the Kuiper belt. This new object is twice as far from the Sun as Neptune and is roughly half the size of Pluto. The body’s highly unusual orbit is difficult to explain using previous theories of the formation of the outer Solar System.
Currently 58 astronomical units from the Sun (1 astronomical unit, or AU, is the distance between the Earth and the Sun), the new object never approaches closer than 50 AU, because its orbit is close to circular. Almost all Kuiper belt objects discovered beyond Neptune are between 30 AU and 50 AU away. Beyond 50 AU, the main Kuiper belt appears to end, and what few objects have been discovered beyond this distance have all been on very high eccentricity (non-circular) orbits. Most of these high-eccentricity orbits are the result of Neptune “flinging” the object outward by a gravitational slingshot. However, because this new object does not approach closer than 50 AU, a different theory is needed to explain its orbit. Complicating the problem, the object’s orbit also has an extreme tilt, being inclined (tilted) at 47 degrees to the rest of the Solar System.
The Discovery and Follow-up
The object, which received the official designation 2004 XR 190 in the International Astronomical Union’s official announcement, was discovered during routine operation of the Canada-France Ecliptic Plane Survey (CFEPS) running as part of the Legacy Survey on the Canada France Hawaii Telescope. For now, the discoverers are using the temporary nickname “Buffy” to identify the new object, although they have proposed a different official name in keeping with normal procedures for naming such objects.
Buffy was extracted from the mountain of Legacy Survey data (about 50 gigabytes per hour of operation) by powerful computers combing through the telescopic images and producing hundreds of candidates. Astronomers then sift through the candidates to identify the distant comets.
Astronomer Lynne Allen of the University of British Columbia was the first to lay eyes on the new object, as she completed the initial identification in the course of processing CFEPS data from December 2004. “It was quite bright compared to the usual Kuiper belt objects we find”, said Dr. Allen, “but what was more interesting was how far away it was.”
The object’s brightness implies it is likely between 500 and 1000 kilometers (300 to 600 miles) in diameter. Buffy is thus a very large Kuiper belt object, but about half a dozen are larger.
“We immediately realized that the object was about twice as far as Neptune from the Sun and that its orbit was potentially nearly circular,” said UBC professor Brett Gladman, who noticed the unusual nature of the object when determining its orbit, “but further observations were required.”
One to two years of observations of a Kuiper belt object are required before their orbits can be precisely measured. The first additional observations of Buffy came in October 2005 when Gladman and Phil Nicholson of Cornell University used the Hale 5-meter telescope to re-observe the object.
Measurement of Buffy’s new position proved that the orbit was not only extremely tilted, inclined (tilted) at 47 degrees to the plane of the planetary system (essentially tying the record for a Kuiper belt object) but confirmed that Buffy was unlike any other previously-known object because it was on a nearly circular orbit while at a very large distance.
More measurements of Buffy’s position on images from telescopes at Kitt Peak National Observatories in Arizona by team members Joel Parker (Southwest Research Institute), as well as JJ Kavelaars (National Research Council of Canada, Herzberg Institute of Astrophysics) and Wes Fraser (University of Victoria), through November 2005 refined the estimate for Buffy’s closest approach to the Sun. Additional observations, to further confirm the orbit, where then provided by the CFHT Legacy Survey project. Astronomers will need to wait until February 2006 to measure the fine details of the Buffy’s orbit.
The team have reported their find to the Minor Planet Center, the clearinghouse for astronomical measurements of new minor planets. “To find the first known object with a nearly circular orbit beyond 50 AU is indeed intriguing,” reacted Brian Marsden, director of the MPC.
Challenging Theories
Although it is neither the smallest, largest, nor most distant object discovered in this region, the new Kuiper belt object has a highly unusual orbit which challenges theories of the evolution of the Solar System.
Why is Buffy’s orbit considered so unusual? Only one other detected object, Sedna, remains further than 50 astronomical units (AUs) from the Sun throughout its entire orbit. However, Sedna is on a very elliptical orbit, swooping in to 76 AU before traveling back out beyond 900 AU. In contrast, Buffy spends all of its time in the narrow range between 52 and 62 AU from the Sun. Combined with the tilt in its orbit, this new object challenges current theories about the history of the early Solar System.
Astronomers have detected other Kuiper belt objects that spend most of their time beyond 50 AU. These are on very elliptical orbits, and almost all approach within 38 AU of the Sun. That close approach places those objects within the reach of the gravitational influence of Neptune. These objects are generally thought to have been scattered out to their present orbits by a gravitational slingshot with Neptune. This group of objects was thus called the “Scattered Disk”.
Prior to the discovery of Buffy, a few other Kuiper belt objects were discovered which spend much of their time beyond 50 AU like those in the “Scattered Disk”, yet did not approach within the gravitational reach of Neptune. This group has been named the “Extended Scattered Disk”. Two of its members are 1995 TL8 and 2000 YW134, which approach to 40 AU of the Sun but have fairly elliptical orbits that take them back out beyond 60 AU. Two more extreme examples of the “Extended Scattered Disk” are 2000 CR105, which approaches to 44 AU, and Sedna, which never comes closer to the Sun than 76 AU.
Due to their large eccentricities, these objects are likely to have been strongly perturbed by something, although it could not have been Neptune because they do not come close enough to be scattered by that planet’s gravitational force. As both Sedna and 2000 CR105 also travel beyond 500 AU from the sun, one theory is that after being scattered by Neptune, a passing star could have pulled their closest approaches away from the Sun.
Buffy is clearly a member of the “Extended Scattered Disk”. However, Buffy’s almost circular orbit makes it stand out from the other members. In addition, Buffy’s large orbital tilt is not so easily explained by the passing star idea. If a star could have affected Buffy so strongly, it should also have disrupted much of the main Kuiper belt as well. Since astronomers do not detect that strong disruption, a more complex theory is needed to explain Buffy’s orbit.
The elusive explanation may lie in side-effects from rearrangements of the Solar System early in its history. One possibility is that as Neptune’s orbit slowly expanded in the young Solar System, complex gravitational interactions could have caused some Kuiper belt orbits to circularize and tilt. While Buffy’s orbit could have been created this way, this theory would not seem to explain 2000 CR105 and Sedna. This new discovery is exciting because it causes us to rethink our understanding of how the Kuiper belt formed.
The Future
Over the last half decade, theories about the formation of our outer Solar System have been pushed to their limits: unusual Kuiper belt objects, like Buffy, which never come close to Neptune yet have high inclination must be explained.
Although theories that explain individual objects exist, reproducing the entire ensemble of known objects with one process poses a difficult challenge to current solar system models. Because the unusual objects, like Buffy, are very rare, astronomers are still scratching the surface of the dark corners of the Kuiper belt. Future large-scale surveys that systematically explore the Kuiper belt are the only way unlock the mysteries of what happened early in the history of our Solar System.
Original Source: Canada-France-Hawaii Telescope