A comet on a comet? That’s what it looks like, but you’re witnessing the most dramatic outburst ever recorded at 67P/Churyumov-Gerasimenko by the Rosetta spacecraft. The brilliant plume of gas and dust erupted on July 29 just two weeks before perihelion.
In a remarkable display of how quickly conditions on a comet can change, the outburst lasted only about 18 minutes, but its effects reverberated for days.
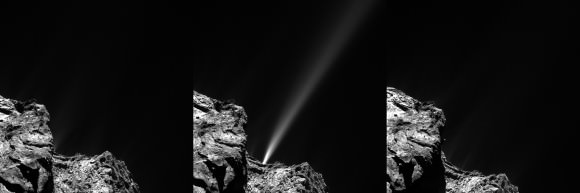
The photos were taken from a distance of 116 miles (186 km) from the center of the comet. Copyright: ESA/Rosetta/MPS for OSIRIS Team MPS/UPD/LAM/IAA/SSO/INTA/UPM/DASP/IDA
In a sequence of images taken by Rosetta’s scientific camera OSIRIS, the brilliant, well-defined jet erupts from the side of the comet’s neck in the Anuket region. It was first seen in a photo taken at 8:24 a.m. CDT, but not in one taken 18 minutes earlier, and had faded significantly in an image captured 18 minutes later. The camera team estimates the material in the jet was traveling at a minimum of 22 mph (10 meters/sec), but possibly much faster.
It’s the brightest jet ever seen by Rosetta. Normally, the camera has to be set to overexpose 67P/C-G’s nucleus to reveal the typically faint, wispy jets. Not this one. You can truly appreciate its brilliance because a single exposure captures both nucleus and plume with equal detail.
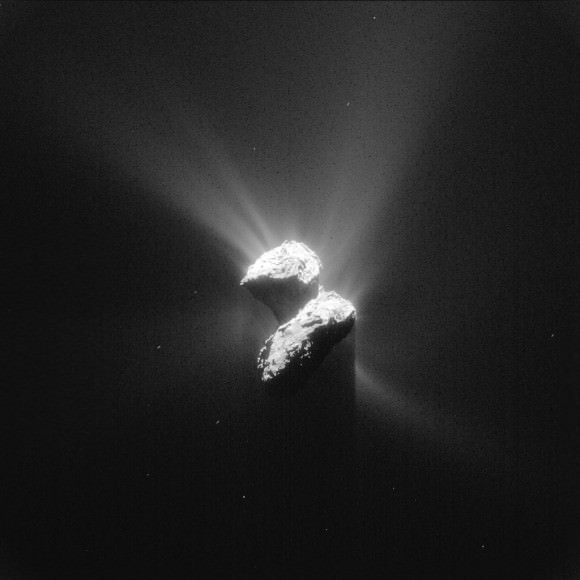
We all expected fireworks as the comet approached perihelion in its 6.5 year orbit around the Sun. Comets are brightest at and shortly after perihelion, when they literally “feel the heat”. Solar radiation vaporizes both exposed surface ices and ice locked beneath the comet’s coal-black crust. Vaporizing subsurface ice can created pressurized pockets of gas that seek a way out either through an existing vent or hole or by breaking through the porous crust and erupting geyser-like into space.
Jets carry along dust that helps create a comet’s fuzzy coma or temporary atmosphere, which are further modified into tails by the solar wind and the pressure of sunlight. When conditions and circumstances are right, these physical processes can build comets, the sight of which can fill the human heart with both terror and wonder.
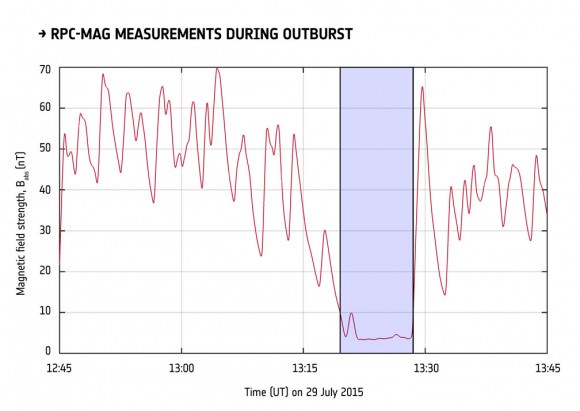
This recent show of activity may be just the start of a round of outbursts at 67P/C-G. While perihelion occurs on this Thursday, a boost in a comet’s activity and brightness often occurs shortly after, similar to the way the hottest part of summer lags behind the date of summer solstice.
Rosetta found that the brief and powerful jet did more than make a spectacle — it also pushed away the solar wind’s magnetic field from around the nucleus as observed by the ship’s magnetometer. Normally, the Sun’s wind is slowed to a standstill when it encounters the gas cloud surrounding the nucleus.
“The solar wind magnetic field starts to pile up, like a traffic jam, and eventually stops moving towards the comet nucleus, creating a magnetic field-free region on the Sun-facing side of the comet called a ‘diamagnetic cavity’,” explained Charlotte Götz, magnetometer team member, on the ESA Rosetta website.
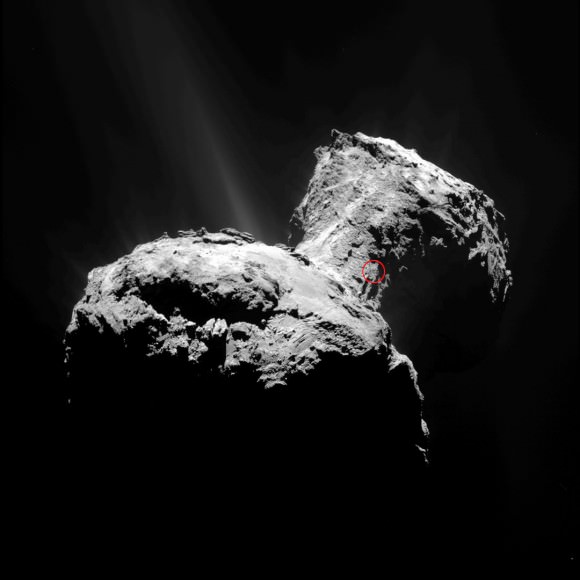
Only once before at Halley’s Comet has a magnetically “empty” region like this been observed. But that comet was so much more active than 67P/C-G and up until July 29, Halley’s remained the sole example. But following the outburst on that day, the magnetometer detected a diamagnetic cavity extending out at least 116 miles (186 km) from the nucleus. This was likely created by the outburst of gas, forcing the solar wind to ‘stop’ further away from the comet and thus pushing the cavity boundary outwards beyond where Rosetta was flying at the time.
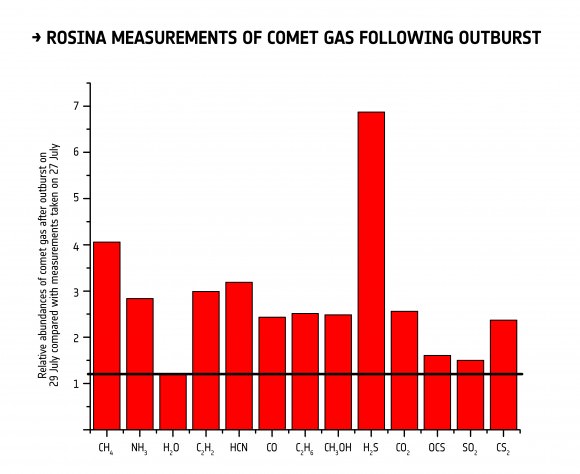
Soon afterward the outburst, the comet pressure sensor of ROSINA detected changes in the structure of the coma, while its mass spectrometer recorded changes in the composition of outpouring gases. Compared to measurements made two days earlier, carbon dioxide increased by a factor of two, methane by four, and hydrogen sulphide by seven, while the amount of water stayed almost constant. No question about it – with all that hydrogen sulfide (rotten egg smell), the comet stunk! Briefly anyway.
It was also more hazardous. In early July, Rosetta recorded and average of 1-3 dust hits a day, but 14 hours after the event, the number leapt to 30 with a peak of 70 hits in one 4-hour period on August 1. Average speeds picked up, too, increasing from 18 mph (8 m/s) to about 45 mph (20 m/s), with peaks at 67 mph (30 m/s). Ouch!
“It was quite a dust party!” said Alessandra Rotundi, principal investigator of GIADA (Grain Impact Analyzer and Dust Accumulator).
67P/C-G’s little party apparently wasn’t enough to jack up its brightness significantly as seen from Earth, but that doesn’t mean future outbursts won’t. We’ll be keeping an eye on any suspicious activity through perihelion and beyond and report back here.