[/caption]
As one of the zodiacal signs, Virgo resides directly on the ecliptic plane and was one of the original 48 constellations charted by Ptolemy. It spans 1294 degrees and is the second largest constellation in the sky. Virgo also contains the point where the celestial equator crosses the ecliptic plane – the the autumn equinox. Between 9 and 15 stars make up its asterism and it contains 96 Bayer Flamsteed designated stars within its confines. Virgo is bordered by the constellations of Bootes, Coma Berenices, Leo, Crater, Corvus, Hydra, Libra and Serpens Caput. It is visible to all observers located at latitudes between +80° and ?80° and is best seen at culmination during the month of May.
There are two annual meteor showers associated with constellation Virgo. The Virginids peak on or about April 10th of each year and will appear to come from a point in the sky near Gamma. This is a relatively active and predictable meteor shower and you can expect to see about 10 meteors per hour on the average during a dark night from a dark location. The second is the Mu Virginids, which peak on or about April 25th. This is also a fairly reliable meteor shower and you can expect to see 7 to 10 meteors per hour on the average coming from an area near the Virgo/Leo border.
In mythology, Virgo is meant to represent the “Virgin”, but who exactly this woman is has never been established – only that she plays an important cultural role. Virgo is often portrayed carrying two sheaves of wheat, one of which is marked by the bright star Alpha – Spica – and it is the only astrological sign represented by a female. Perhaps she is Astraea, the virgin daughter of Zeus who was known as the goddess of justice. After all, Libra, the scales of justice is nearby!
Let’s begin our tour of Virgo with its brightest star – Alpha – the “a” symbol on our map. Alpha Viriginis is best known as Spica. Located 262 light-years away from Earth, 1.0 magnitude Spica glows with the combined light of four unresolved stars and has a visual luminosity 2100 times that of the Sun. As a rotating ellipsoidal variable, the four stars cause complex changes in luminosity by distorting the shape of the brightest components. The dominant star – Spica A – has a mass 11 times that of the Sun and fluctuates in physical size as it varies in brightness. The primary star is at maximum when smallest, giving it the highest photospheric surface temperature. Spica B has a mass of 7 suns. As a spectral type B, these two components produce more light in ultraviolet due to exceedingly high surface temperatures. Spica has two distant telescopic companions – magnitude 12 to the north-northeast, and magnitude 10.5 to the east-northeast.
Now head towards Beta – the “B”. Named Zavijava (sounds like something you’d get at Starbuck’s doesn’t it?) and located about 36 light years away from our solar system, this star holds a very special place in history because of its position in the sky. Since it is so near the ecliptic plane, it can frequently be occulted by the Moon, occasionally a planet, and even the Sun. In Zavijava’s case, it had the honor of being the star Einstein used during the solar eclipse of September 21, 1922 to determine the speed of light in space! What’s more, according to studies, Beta Virginis could host two or three Jupiter-sized planets – either brown dwarf stars in wide orbits or true planetary objects.
Ready for Gamma Virginis? That’s the “Y” symbol. Best known as Porrima, this binary star of nearly matched magnitudes was an easy object for amateur astronomers, but now the smaller apparent distance between the stars requires a larger telescope. Because of its relatively quick orbital period of 168.93 years, you’ll sometimes hear Porrima referred to as the “Shrinking Star”. At the time of this writing (early 2009), the pair is only separated by about .04″ and it will be another 11 years before they have moved apart enough again to be easily split with a small telescope!
Because there are massive amounts of deep sky objects in Virgo, annotating a map would be so cluttered it would be difficult to read. Let us begin first with the chart we have above which highlights the brighter objects in Virgo – ones easily seen with binoculars and small telescopes. Ready to dance?
Our first target will be Messier 104 (RA 12 : 40.0 Dec -11 : 37). Now, shake your fist at Spica… Because that’s all it takes to find the awesome M104, eleven degrees due west. (If you still have trouble finding M104, don’t worry. Try this trick! Look for the upper left hand star in the rectangle of Corvus – Delta. Between Spica and Delta is a diamond-shaped pattern of 5th magnitude stars. Aim your scope or binoculars just above the one furthest south.) Also known as the “Sombrero Galaxy” this gorgeous 8th magnitude spiral galaxy was discovered by Pierre Mechain in 1781, added by hand to Messier’s catalog and observed independently by William Herschel as H I.43 – who was probably the first to note its dark inclusion. The Sombrero’s rich central bulge is comprised of several hundred globular clusters and can be hinted at in just large binoculars and small telescopes. Large aperture telescopes will revel in this galaxy’s “see through” qualities and bold, dark dustlane – making it a seasonal favorite!
Now, let’s take a look at one of the brightest members of the Virgo Cluster – Messier 49. Located about eight degrees northwest of Delta Virginis almost directly between a pair of 6th magnitude stars (RA 12 29 46 Dec +07 59 59), the giant elliptical galaxy M49 holds the distinction of being the first galaxy in the Virgo cluster to be discovered – and only the second beyond our local group. At magnitude 8.5, this type E4 galaxy will appear as an evenly illuminated egg shape in almost all scopes, and as a faint patch in binoculars. While a possible supernova event occurred in 1969, don’t confuse the foreground star noted by Herschel with something new! Although most telescopes won’t be able to pick this region apart, there are also many fainter companions near M49, including NGC 4470. But a sharp-eyed observer named Halton Arp noticed them and listed them as Peculiar Galaxy 134 – one with “fragments!”
Next up, Messier 87 (RA 12 : 30.8 Dec +12 : 24). It’s a radio-source galaxy so bright it can be seen in binoculars – 8.6 magnitude M87, about two fingerwidths northwest of Rho Virginis. This giant elliptical galaxy was discovered by Charles Messier in 1781 and cataloged as M87. Spanning 120,000 light-years, it’s an incredibly luminous galaxy containing far more mass and stars than the Milky Way Galaxy – gravitationally distorting its four dwarf satellites galaxies. M87 is known to contain in excess of several thousand globular clusters – up to 150,000 – and far more than our own 200.
In 1918, H. D. Curtis of Lick Observatory discovered something else – M87 has a jet of gaseous material extending from its core and pushing out several thousand light-years into space. This highly perturbed jet exhibits the same polarization as synchrotron radiation – a property of neutron stars. Containing a series of small knots and clouds as observed by Halton Arp at Palomar in 1977, he also discovered a second galaxy jet in 1966 erupting in the opposite direction. Thanks to these two properties, M87 made Arp’s “Catalog of Peculiar Galaxies” as number 152. In 1954 Walter Baade and R. Minkowski identified M87 with radio source Virgo A, discovering a weaker galactic halo in 1956. Its position over an x-ray cloud extending through the Virgo cluster make M87 a source of an incredible amount of x-rays. Because of its many strange properties, M87 remains a target of scientific investigation. The Hubble Space Telescope has shown a violent nucleus surrounded by a fast rotating accretion disc, whose gaseous make-up may be part of a huge system of interstellar matter. As of today, only one supernova event has been recorded – yet M87 remains one of the most active and highly prized study galaxies of all. Capture it tonight!
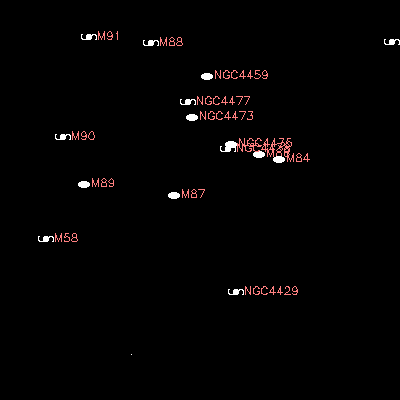
Now we’re heading for our more detailed map and the galaxy fields of Virgo about four fingerwidths east-southeast of Beta Leonis. As part of Markarian’s Chain, this set of galaxies can all be fitted within the same field of view with a 32mm eyepiece and a 12.5″ scope, but not everyone has the same equipment. Set your sights toward M84 and M86 and let’s discover!
Good binoculars and small telescopes reveal this pair with ease as a matched set of elliptical galaxies. Mid-sized telescopes will note the western member of the pair – M84 – is seen as slightly brighter and visibly smaller. To the east and slightly north is larger M86 – whose nucleus is broader, and less intensely brilliant. In a larger scope, we see the galaxies literally “leap” out of the eyepiece at even the most modest magnifications. Strangely though, additional structure fails to be seen. As aperture increases, one of the most fascinating features of this area becomes apparent. While studying the bright galactic forms of M84/86 with direct vision, aversion begins to welcome many other mysterious strangers into view. Forming an easy triangle with the two Messiers and located about 20 arc-minutes south lies NGC 4388. At magnitude 11.0, this edge-on spiral galaxy has a dim star-like core to mid-sized scopes, but a classic edge-on structure in larger ones.
At magnitude 12, NGC 4387 is located in the center of a triangle formed by the two Messiers and NGC 4388. NGC 4387 is a dim galaxy – hinting at a stellar nucleus to smaller telescopes, while the larger ones will see a very small face-on spiral galaxy with a brighter nucleus. Just a breath north of M86 is an even dimmer patch of nebulosity – NGC 4402 – which needs higher magnifications to be detected in smaller scopes. Large apertures at high power reveal a noticeable dust lane. The central structure forms a curved “bar” of light. Luminosity appears evenly distributed end to end, while the dust lane cleanly separates the central bulge of the core. East of M86 are two brighter NGC galaxies – 4435 and 4438. Through average scopes, NGC 4435 is easily picked out at low power with a simple star-like core and wispy round body structure. NGC 4438 is dim, but even large apertures make elliptical galaxies a bit boring. The beauty of NGC 4435 and NGC 4438 is simply their proximity to each other. 4435 shows true elliptical structure, evenly illuminated, with a sense of fading toward the edges… But 4438 is quite a different story! This elliptical galaxy is much more elongated. A highly conspicuous wisp of galactic material can be seen stretching back toward the brighter, nearby galaxy pair M84/86.
Ready for bright galaxy Messier 58 (RA 12 : 37.7 Dec +11 : 49)? It’s a spiral galaxy actually discovered by Messier in 1779! As one of the brightest galaxies in the Virgo cluster, M58 is one of only four that have barred structure. It was cataloged by Lord Rosse as a spiral in 1850. In binoculars, it will look much like our previously studied ellipticals, but a small telescope under good conditions will pick up the bright nucleus and a faint halo of spiral galaxy structure – while larger ones will see the central concentration of the bar across the core. Chalk up another Messier study for both binoculars and telescopes and let’s get on to something really cool!
Around a half degree southwest are NGC 4567 and NGC 4569. L. S. Copeland dubbed them the “Siamese Twins,” but this galaxy pair is also considered part of the Virgo cluster. While seen from our viewpoint as touching galaxies, no evidence exists of tidal filaments or distortions in structure, making them a line of sight phenomenon and not interacting members. While that might take little of the excitement away from the “Twins,” a supernova event has been spotted in NGC 4569 as recently as 2004. While the duo is visible in smaller scopes as two, with soft twin nuclei, intermediate and large telescopes will see an almost V-shaped or heart-shaped pattern where the structures overlap. If you’re doing double galaxy studies, this is a fine, bright one! If you see a faint galaxy in the field as well, be sure to add NGC 4564 to your notes. Moving about a degree north will call up face-on spiral galaxy M89, which will show a nice core region in most telescopes. One half degree northeast is where you will find the delightful 9.5 magnitude M90 – whose dark dust lanes will show to larger telescopes.
Virgo contains many, many more fine objects – so be sure to get a detailed star chart and spend some time with this great constellation!
Sources:
Wikipedia
SEDS
Chandra Observatory
Charts Courtesy of Your Sky.