There is nothing in the Universe more awe inspiring or mysterious than a black hole. Because of their massive gravity and ability to absorb even light, they defy our attempts to understand them. All their secrets hide behind the veil of the event horizon.
What do they look like? We don’t know. They absorb all the radiation they emit. How big are they? Do they have a size, or could they be infinitely dense? We just don’t know. But there are a few things we can know. Like how massive they are, and how fast they’re spinning.
Wait, what? Spinning?
Consider the massive star that came before the black hole. It was formed from a solar nebula, gaining its rotation by averaging out the momentum of all the individual particles in the cloud. As mutual gravity pulled the star together, through the conservation of angular momentum it rotated more rapidly. When a star becomes a black hole, it still has all that mass, but now compressed down into an infinitesimally smaller space. And to conserve that angular momentum, the black hole’s rate of rotation speeds up… a lot.The entire history of everything the black hole ever consumed, averaged down to a single number: the spin rate.
If the black hole could shrink down to an infinitely small size, you would think that the spin rate might increase to infinity too. But black holes have a speed limit.
“There is a speed limit to the spin of a black hole. It’s sort of set by the faster a black hole spins, the smaller is its event horizon.”
That’s Dr. Mark Morris, a professor of astronomy at UCLA. He has devoted much of his time to researching the mysteries of black holes.
“There is this region, called the ergosphere between the event horizon and another boundary, outside. The ergosphere is a very interesting region outside the event horizon in which a variety of interesting effects can occur.”
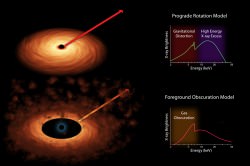
Imagine the event horizon of a black hole as a sphere in space, and then surrounding this black hole is the ergosphere. The faster the black hole spins, the more this ergosphere flattens out.
“The speed limit is set by the event horizon, eventually, at a high enough spin, reaches the singularity. You can’t have what’s called a naked singularity. You can’t have a singularity exposed to the rest of the Universe. That would mean that the singularity itself could emit energy or light and somebody outside could actually see it. And that can’t happen. That’s the physical limitation of how fast it can spin. Physicists use units for angular momentum that are cast in terms of mass, which is a curious thing, and the speed limit can be described as the angular momentum equals the mass of the black hole, and that sets the speed limit.”
Just imagine. The black hole spins up to the point that it’s just about to reveal itself. But that’s impossible. The laws of physics won’t let it spin any faster. And here’s the amazing part. Astronomers have actually detected supermassive black holes spinning at the limits predicted by these theories.
One black hole, at the heart of galaxy NGC 1365 is turning at 84% the speed of light. It has reached the cosmic speed limit, and can’t spin any faster without revealing its singularity.
The Universe is a crazy place.