Elon Musk has been rather outspoken in recent years about his plan to create a human settlement on Mars. Stressing the need for a “backup location” for humanity, he has dedicated his company (SpaceX) to the creation of a reusable spacecraft (aka. the Interplanetary Transport System) that in the coming decades will be able to transport one-hundred people at a time to Mars.
In addition to Musk, Dutch entrepreneur Bas Lansdorp has also expressed an interest in creating a permanent settlement on Mars. In 2012, he founded MarsOne with the intent of developing the necessary expertise to mount one-way trips to the Red Planet by 2032. And according to an announcement from the government of Dubai, it seems they aren’t the only ones looking to colonize the Red Planet.
The announcement came on February 14th, 2017, during the 5th World Government Summit – which was held this year in Dubai. In the midst of presentations by notaries like Ban-Ki-Moon, Elon Musk, and Barack Obama, Sheikh Mohammed bin Rashid Al Maktoum and Sheikh Mohamed bin Zayed Al Nahyan shared their country’s vision of putting 600,000 humans on the Red Planet by the next century – known as the “Mars 2117 Project”.
In the course of his speech, Sheikh Mohammed emphasized the UAEs commitment to space sciences and its desire to accomplish one of the longest-held dreams of humanity:
“Human ambitions have no limits, and whoever looks into the scientific breakthroughs in the current century believes that human abilities can realize the most important human dream. The new project is a seed that we plant today, and we expect future generations to reap the benefits, driven by its passion to learn to unveil a new knowledge. The landing of people on other planets has been a longtime dream for humans. Our aim is that the UAE will spearhead international efforts to make this dream a reality.”
As growing investors in the field of space research, Sheikh Mohammed indicated that this project will accelerate the UAE’s commitment in this regard. Recent accomplishments by the Emirati space program include the successful deployment of the UAE’s first nanosatellite – Nayif-1 – which was launched a day after the Mars 2117 announcement (Feb. 15th, 2017).
This nanosatellite was the result of collaborative work between the Mohammed bin Rashid Space Centre (MBRSC) and the American University of Sharjah (AUS). Its intended purpose is to provide opportunities and hands-on experience for Emirati engineering students, as well as developing expertise in the designing, building, testing and operating of nanosatellites.
And then there’s the Hope Spacecraft, a project which was commissioned in 2015 by the Emirates Mars Mission. This project calls for the creation of a compact, hexagonal spacecraft that will reach the Red planet by 2021 and spend the next two years studying its atmosphere and weather. Not only is this mission designed to provide the first truly global picture of the Martian atmosphere, it will also be the first orbiter deployed by an Arab country.
Meanwhile, Sheikh Mohamed bin Zayed – the Crown Prince of Abu Dhabi and the Deputy Supreme Commander of the UAE Armed Forces – said that the objective of the project is to develop the skills and capacities of the UAE’s space program. He also indicates that the project will benefit research institutions and advance the fields of transportation, energy and food production here on Earth.
The “Mars 2117” project will develop an Emirati and international team of scientists to push the human exploration of Mars in years to come. pic.twitter.com/5ujxvyC8As
— HH Sheikh Mohammed (@HHShkMohd) February 14, 2017
“Mars 2117” is a seed we are sowing today to reap the fruit of new generations led by a passion for science and advancing human knowledge. pic.twitter.com/IExtnpiO2B
— HH Sheikh Mohammed (@HHShkMohd) February 14, 2017
“The Mars 2117 Project is a long term project, where our first objective is to develop our educational system so our sons will be able to lead scientific research across the various sectors,” he said. “The UAE became part of a global scientific drive to explore space, and we hope to serve humanity through this project.”
Elements of the project were showcased at the Summit by a team of Emirati engineers, scientists and researchers – which included a concept for a human city that would be built by robots. It also showcased aspects of the inhabitants’ lifestyle, like the transportation they would use, how they would generate power, how they would grow food, the infrastructure they would build, and the materials that would be used to construct the colony.
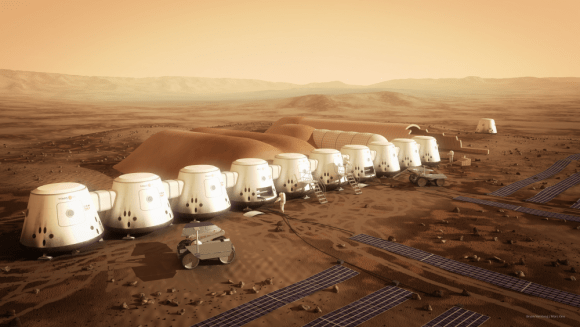
Given the long-term nature of this project, it will be broken down into multiple phases that will take place over the next few decades. Phase One will focus on preparing the scientists who will attempt to address all the challenges and concerns of sending human beings on a one-way trip to Mars. At the same time, the project calls for the creation of an Emiratis science team that will work with the international scientific community to speed up the research efforts.
Particular areas of concern will include creating spacecraft that are fast enough to ferry people to and from Earth in a respectable time frame. Then there’s the task of creating a working model of what the settlement will look like, and how the needs of its inhabitants will be met. Naturally, this will include methods for growing food and seeing to the health, safety, transportation, and energy needs of the colonists.
In the future, the UAE also anticipates that uncrewed missions will be mounted to explore the surface of Mars and locate a possible site for the future colony. In short, they are not only joining the “Mars or Bust” club, but also the international community of space explorers.
Further Reading: Government of Dubai