When it comes to the future of space exploration, one of the biggest questions is, “how and when will we travel to the nearest star?” And while space agencies have been pondering this question and coming up with proposals for decades, none of them have advanced beyond the theory stage. For the most part, their efforts has been focused on possible missions to Mars and the outer Solar System.
But there are some people, like Dr. Gerald Jackson, who are working towards making an interstellar mission possible in the near future. He and his research team, which have been funded by NASA in the past, are looking to create an antimatter engine that will be capable of reaching (or exceeding) 5% the speed of light. Towards this end, they have launched a Kickstarter campaign to fund their efforts.
As advanced propulsion concepts go, antimatter has quite a lot going for it. As propulsion goes, it has the highest specific energy of any known method, 100 times more than fission/fusion reactions, and 10 billion times more than chemical propellants. It is also the most fuel-efficient, requiring mere milligrams of antimatter to produce the same amount of energy as tons of chemical fuel.
Typically, this theoretical concept relies on the collision between hydrogen and antihydrogen (which have the same mass but opposite charge) to generate thrust. This process unleashes energy and a shower of particles (pions and muons), which can be channeled by a magnetic nozzle to generate thrust.
And while laboratories like CERN have been producing antimatter, and research is being conducted on large-scale storage, no propulsion systems exist that could turn antimatter into thrust. Dr. Jackson, a graduate of Cornell University, is hoping to change that. Before entering the private sector, Jackson worked as an accelerator physicist at the Fermi National Accelerator Laboratory for 14 years.
In 2002, he co-founded a limited-liability company (HBar Technologies) for the sake of developing commercial markets for antimatter. In 2002, NASA’s Institute for Advanced Concepts (NIAC) awarded Dr. Jackson and his company $75,000 to develop a mission concept that could traverse 250 AUs of space within 10 years time, and with a fuel supply of 10 kg.
These specifications essentially called for the creation of an antimatter rocket that could travel as far as the heliopause within a decade’s time. The result was a propulsion concept that relied on a beam that would fire focused antiprotons onto a sail to generate propulsion. This sail would measure 5 meters in diameter and be composed of a carbon backing on one side and uranium foil on the other (measuring 15 and 296 microns thick, respectively).
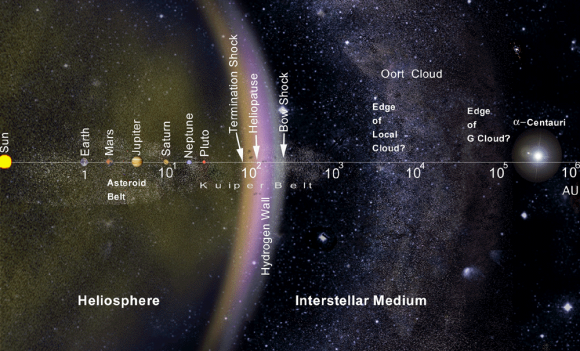
When a pulse of antiprotons is annihilated against a small section of the uranium side, the resulting fission causes momentum. As Dr. Jackson explained to Universe Today via email:
“Note that antiprotons have a negative electrical charge, similar to an electron. When the antiprotons enter the sail, they displace an electron orbiting an uranium nucleus. Because antiprotons and electrons do not share any quantum numbers, the antiproton immediately cascades down into the atomic ground state, causing a high probability of interaction between the antiproton and either a proton or neutron within the nucleus.
“On average, a fission event results in the creation of two daughter nuclei of roughly equal mass. These daughters travel in opposite directions with a kinetic energy of 1 MeV per proton or neutron. Because the daughters are charged, the one travelling further into the sail is absorbed and transfers is forward momentum. The other daughter flies into space with an exhaust velocity of 4.6% of lightspeed. This selective transfer of momentum is thrust.”
Unfortunately, due to the budget environment of the time, the NIAC was forced to cancel its funding after a second round had been granted. Because of this, Dr. Jackson and his colleagues are now seeking public support so that they may finish their work on the experimental sail and prepare it for exposure to an antiproton beam.
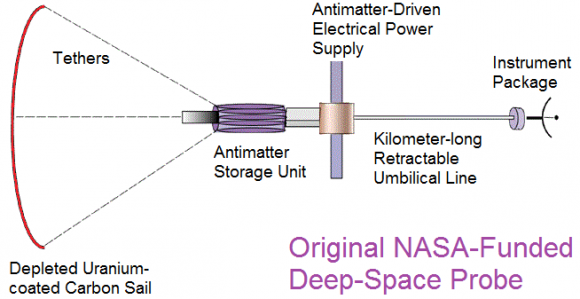
Much like Project Starshot (whom they acknowledge on their campaign page), Jackson and his team are looking to produce an interstellar mission proposal that does not involve shortcuts (i.e. warp drive, wormholes, star gates, etc.). Starshot, as you may recall, calls for a wafer craft and a laser-driven lightsail that would be capable of reaching speeds of up to 20% the speed of light, thus making the journey to Alpha Centauri in 20 years.
In the same vein, a antiproton-driven sail that could reach speeds of 5% the speed of light or more would be capable of making it to Alpha Centauri (or Proxima Centauri) in about 90 years time. All the while, the science behind it would remain within the realm of established physics, being consistent with Newton’s Laws of Motion and Einstein’s Theory of Special Relativity.
“The revolutionary aspect of the antimatter-driven sail is that the antimatter is not the fuel, but rather the spark plug that initiates fission reactions,” said Jackson. “Because the fission reactions can produce thrust without heavy shielding or other structures, the mass of the propulsion system can be comparable to the mass of the instrument package.”
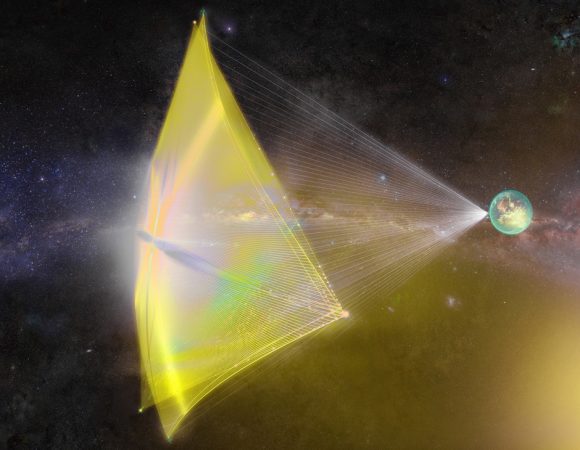
To see their project through, Jackson and his colleagues are hoping to raise $200,000. Should they prove successful, they hope to mount follow-up campaigns to finance a series of validation experiments, storage demonstrations, and mission details. In the end, their goal is nothing less than making antimatter propulsion a reality, which they hope will one day lead interstellar mission.
“We expect that these campaigns will provide the data needed to convince people to fund full scale antimatter production and an actual mission to a nearby solar system,” Jackson added. “The goal of those early interstellar missions is to provide information about these other solar systems, such as whether they are habitable or inhabited. If the latter, we will want to study or interact with those life forms in follow-on missions. If habitable and not inhabited, we need sufficient information to assure the success of a manned migratory mission.”
As of the penning of this article, Jackson and his colleagues have raised $672 of their $200,000 goal. However, the campaign launched only a few days ago and will remain open for another 25 days. For those interesting in following their progress, or have an interest in donating to their cause, check out the links below.
Further Reading: Kickstarter, Antima:tterDrive