In 2003, the Japanese Aerospace Exploration Agency (JAXA) launched the Hayabusa probe. Its mission was to rendezvous with asteroid 25143 Itokawa in 2005. Once there, it studied a number of things about Itokawa, including its shape, topography, composition, colour, spin, density, and history. But the most exciting part of its mission was to collect samples from the asteroid and return them to Earth.
The mission suffered some complications, including the failure of Minerva, Hayabusa’s detachable mini-lander. But Hayabusa did land on the asteroid, and it did collect some samples; tiny grains of material from the surface of Itokawa. This was the first time a mission had landed somewhere and returned samples, other than missions to the Moon.
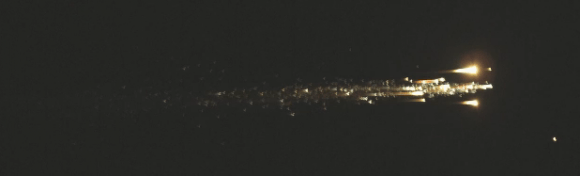
Once the collected grains made it back to Earth in 2010, and were confirmed to be from the asteroid, scientists got excited. These grains would be key to helping understand the early Solar System when the planetary bodies were formed. And they have revealed a sometimes violent history going back 4.5 billion years.
The grains themselves are truly microscopic, at just over 10 micrometers in size. The marks and surface patterns on them are measured in nanometers. Initially, all the marks on the surfaces of the particles were thought to be of one type. But the team behind the study used electron microscopes and X-Ray Microtomography to reveal four different types of patterns on their surfaces.
One 4.5 billion year old pattern shows crystallization from intense heat. At this time period, Itokawa was part of a larger asteroid. The second pattern indicates a collision with a meteor about 1.3 billion years ago. Another pattern was formed by exposure to the solar wind between 1 million and 1,000 years ago. A fourth pattern detected by scientists shows that the particles have been rubbing against each other.
The team has concluded that Itokawa didn’t always exist in its current shape and form. When it was formed over 4 billion years ago, it was about 40 times bigger than it is now. That parent body was destroyed, and the researchers think that Itokawa re-formed from fragments of the parent body.
If there is still any lingering doubt about the violent nature of the Solar System’s history, the grains from Itokawa help dispel it. Collision, fragmentation, bombardments, and of course solar wind, seem to be the norm in our Solar System’s history.
The return of these samples was a bit of a happy accident. The sample collection mechanism on Hayabusa suffered a failure, and the returned dust grains were actually kicked up by the landing of the probe, and some ended up in the sample capsule.
For their part, JAXA has already launched Hayabusa’s successor, Hayabusa 2. It was launched in December 2014, and is headed for asteroid 162173 Ryugu. It should reach its destination in July 2018, and spend a year and a half there. Hayabusa 2 is also designed to collect asteroid samples and return them to Earth, this time using an explosive device to dig into the asteroid’s surface for a sample. Hayabusa 2 should return to Earth in December 2020.
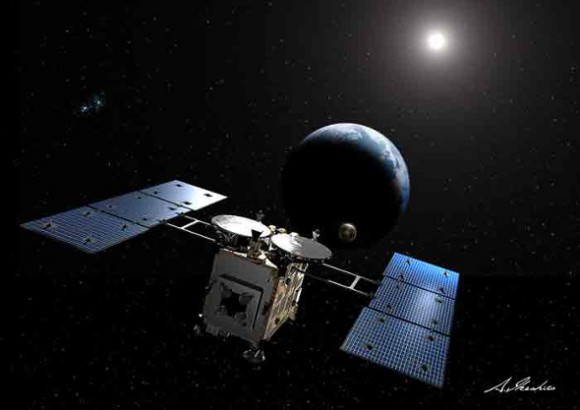
Hayabusa suffered several failures, including the failure of its mini-lander, problems with sample collection, and it even suffered damaged to its solar panels caused by a solar flare, which reduced its power and delayed its arrival at Itokawa. Yet it still ended up being a success in the end.
If Hayabusa 2 can avoid some of these problems, who knows what we may learn from more intentional samples. Sample missions are tricky and complex. If Hayabusa can return samples, it would be only the fourth body to have samples successfully returned to Earth, including the Moon, asteroid Itokawa, and comet Wild 2.