Dr. Stephen Hawking delivered a disturbing theory in 1974 that claimed black holes evaporate. He said black holes are not absolutely black and cold but rather radiate energy and do not last forever. So-called “Hawking radiation” became one of the physicist’s most famous theoretical predictions. Now, 40 years later, a researcher has announced the creation of a simulation of Hawking radiation in a laboratory setting.
The possibility of a black hole came from Einstein’s theory of General Relativity. Karl Schwarzchild in 1916 was the first to realize the possibility of a gravitational singularity with a boundary surrounding it at which light or matter entering cannot escape.
This month, Jeff Steinhauer from the Technion – Israel Institute of Technology, describes in his paper, “Observation of self-amplifying Hawking radiation in an analogue black-hole laser” in the journal Nature, how he created an analogue event horizon using a substance cooled to near absolute zero and using lasers was able to detect the emission of Hawking radiation. Could this be the first valid evidence of the existence of Hawking radiation and consequently seal the fate of all black holes?
This is not the first attempt at creating a Hawking radiation analogue in a laboratory. In 2010, an analogue was created from a block of glass, a laser, mirrors and a chilled detector (Phys. Rev. Letter, Sept 2010); no smoke accompanied the mirrors. The ultra-short pulse of intense laser light passing through the glass induced a refractive index perturbation (RIP) which functioned as an event horizon. Light was seen emitting from the RIP. Nevertheless, the results by F. Belgiorno et al. remain controversial. More experiments were still warranted.
The latest attempt at replicating Hawking radiation by Steinhauer takes a more high tech approach. He creates a Bose-Einstein condensate, an exotic state of matter at very near absolute zero temperature. Boundaries created within the condensate functioned as an event horizon. However, before going into further details, let us take a step back and consider what Steinhauer and others are trying to replicate.
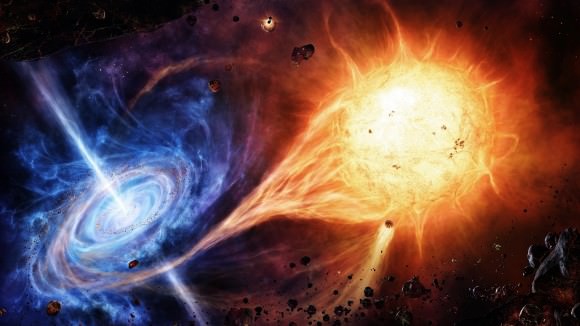
The recipe for the making Hawking radiation begins with a black hole. Any size black hole will do. Hawking’s theory states that smaller black holes will more rapidly radiate than larger ones and in the absence of matter falling into them – accretion, will “evaporate” much faster. Giant black holes can take longer than a million times the present age of the Universe to evaporate by way of Hawking radiation. Like a tire with a slow leak, most black holes would get you to the nearest repair station.
So you have a black hole. It has an event horizon. This horizon is also known as the Schwarzchild radius; light or matter checking into the event horizon can never check out. Or so this was the accepted understanding until Dr. Hawking’s theory upended it. And outside the event horizon is ordinary space with some caveats; consider it with some spices added. At the event horizon the force of gravity from the black hole is so extreme that it induces and magnifies quantum effects.
All of space – within us and surrounding us to the ends of the Universe includes a quantum vacuum. Everywhere in space’s quantum vacuum, virtual particle pairs are appearing and disappearing; immediately annihilating each other on extremely short time scales. With the extreme conditions at the event horizon, virtual particle and anti-particles pairs, such as, an electron and positron, are materializing. The ones that appear close enough to an event horizon can have one or the other virtual particle zapped up by the black holes gravity leaving only one particle which consequently is now free to add to the radiation emanating from around the black hole; the radiation that as a whole is what astronomers can use to detect the presence of a black hole but not directly observe it. It is the unpairing of virtual particles by the black hole at its event horizon that causes the Hawking radiation which by itself represents a net loss of mass from the black hole.
So why don’t astronomers just search in space for Hawking radiation? The problem is that the radiation is very weak and is overwhelmed by radiation produced by many other physical processes surrounding the black hole with an accretion disk. The radiation is drowned out by the chorus of energetic processes. So the most immediate possibility is to replicate Hawking radiation by using an analogue. While Hawking radiation is weak in comparison to the mass and energy of a black hole, the radiation has essentially all the time in the Universe to chip away at its parent body.
This is where the convergence of the growing understanding of black holes led to Dr. Hawking’s seminal work. Theorists including Hawking realized that despite the Quantum and Gravitational theory that is necessary to describe a black hole, black holes also behave like black bodies. They are governed by thermodynamics and are slaves to entropy. The production of Hawking radiation can be characterized as a thermodynamic process and this is what leads us back to the experimentalists. Other thermodynamic processes could be used to replicate the emission of this type of radiation.
Using the Bose-Einstein condensate in a vessel, Steinhauer directed laser beams into the delicate condensate to create an event horizon. Furthermore, his experiment creates sound waves that become trapped between two boundaries that define the event horizon. Steinhauer found that the sound waves at his analogue event horizon were amplified as happens to light in a common laser cavity but also as predicted by Dr. Hawking’s theory of black holes. Light escapes from the laser present at the analogue event horizon. Steinhauer explains that this escaping light represents the long sought Hawking radiation.
Publication of this work in Nature underwent considerable peer review to be accepted but that alone does not validate his findings. Steinhauer’s work will now withstand even greater scrutiny. Others will attempt to duplicate his work. His lab setup is an analogue and it remains to be verified that what he is observing truly represents Hawking radiation.
References:
“Observation of self-amplifying Hawking radiation in an analogue black-hole laser“, Nature Physics, 12 October 2014
“Hawking Radiation from Ultrashort Laser Pulse Filaments”, F. Belgiorno, et al., Phys. Rev. Letter, Sept 2010
“Black hole explosions?”, S. W. Hawking, et al., Nature, 01 March 1974
“The Quantum Mechanics of Black Holes”, S. W. Hawking, Scientific American, January 1977