Join Universe Today in celebrating the 45th anniversary of Apollo 13 with insights from NASA engineer Jerry Woodfill as we discuss various turning points in the mission.
The final scenes of the movie Apollo 13 depict the spacecraft’s dramatic reentry into Earth’s atmosphere. As the seconds count beyond the time radio blackout should have lifted, the Capcom calls for Apollo 13’s crew to answer, but there is no response.
Everyone’s thoughts run through the possibilities: Had the heat shield been compromised by shrapnel from the exploded oxygen tank? Had the previously finicky hatch failed at this critical time? Had the parachutes turned to blocks of ice? Had the Inertial Measurement Unit (IMU) gyros failed, having inadequate time to warm-up causing the capsule to skip off the atmosphere, or incinerate with the crew in a fiery death plunge to Earth?
Of course, the crew finally did answer, but confirmation that Lovell, Haise and Swigert had survived reentry came nearly a minute and a half later than expected.
Some might feel director Ron Howard may have over-sensationalized the re-entry scenes for dramatic effect. But in listening to the actual radio communications between Mission Control and the ARIA 4 aircraft that was searching for a signal from the Apollo 13 crew, the real drama is just as palpable – if not more — than in the movie.
For virtually every reentry from Mercury through Apollo 12, the time of radio blackout was predictable, almost to the second. So why did Apollo 13’s radio blackout period extend for 87 seconds longer than expected, longer than any other flight?
During the Apollo era, the radio blackout was a normal part of reentry. It was caused by ionized air surrounding the command module during its superheated reentry through the atmosphere, which interfered with radio waves. The radio blackout period for the space shuttle program ended in 1988 when NASA launched the Tracking and Data Relay Satellite System (TDRS), which allowed nearly constant communication between the spacecraft and Mission Control.
It is difficult to find official NASA documentation about the extended radio blackout time for Apollo 13. In the mission’s Accident Review Board Report, there’s no mention of this anomaly. The only discussion of any communication problem comes in a section about reentry preparations, after the Service Module was jettisoned. There was a half-hour period of very poor communications with the Command Module due to the spacecraft being in a poor attitude with the Lunar Module still attached. Some of the reentry preparations were unnecessarily prolonged by the poor communications, but was more of a nuisance than an additional hazard to the crew, the report said.
In numerous interviews that I’ve done and listened to in preparation for this series of articles, when those involved with the Apollo 13 mission are asked about why the blackout period was longer than normal, the answer normally comes as a hedged response, with the crew or a flight director indicating they don’t know exactly why it happened. It seems analysis of this has defied a reasonable and irrefutable scientific explanation.
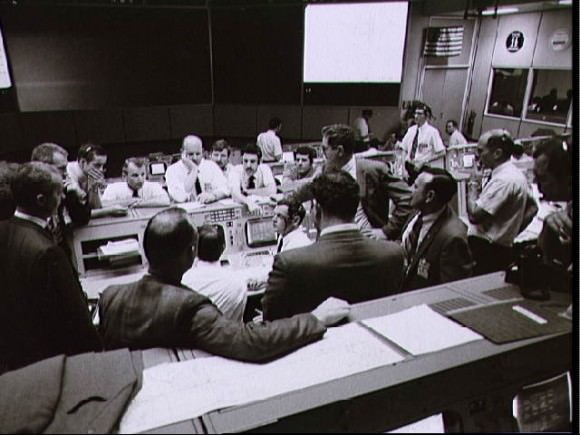
At an event at the Smithsonian Air & Space Museum in 2010, Apollo 13 Flight Director Gene Kranz said he never heard an answer or explanation that he believed, and Fred Haise chuckled and said, “We just did Ron Howard a favor!”
Jim Lovell gave the most detailed response – which is the one most often given as a likely explanation — suggesting it perhaps had to do with a shallowing reentry angle problem, with a strange space-like breeze that seemed to be blowing the spacecraft off-course with respect to entry.
“I think the reason why it was longer was the fact we were coming in shallower than we had planned,” Lovell said at the 2010 event. “Normally we come in from a Moon landing and have to hit the atmosphere inside a very narrow pie-shaped wedge and I think we were continually being pushed off that wedge. The reason was, we found out about 2-3 months after from analysis, was the lander’s venting of cooling vapor. The way we cool the electronic systems in LM was to pass water through a heat exchanger, and that water evaporates into space. That evaporation — which would be insignificant during a normal lunar landing mission — was going on for the 4 days we were using the LM as a lifeboat, acting as a small force, forcing us off the initial trajectory.”
Coming in on a shallower trajectory would result in a longer period in the upper atmosphere where there was less deceleration of the spacecraft. In turn, the reduced pace of deceleration lengthened the time that the heat of reentry produced the ionized gasses that would block communications.
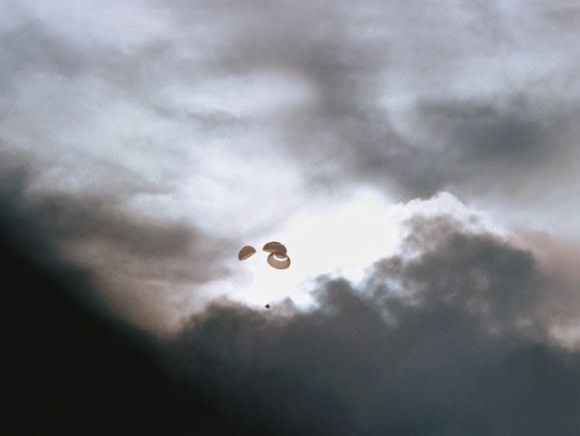
But NASA engineer Jerry Woodfill offers additional insight into the communication delays. He recently spoke with Jerry Bostick, the Flight Dynamics Officer (FIDO) for Apollo 13, who told him, “Many believe the added time resulted from the communication signal skipping, like a stone, over layers of the upper atmosphere because of the shallow entry angle.”
“Bostick likened the radio signals to a stone skipping on a pond, and finally, the signal found a location to sink Earthward,” Woodfill said.
However, this explanation too, leaves questions. Woodfill said he has studied the “signal skipping” phenomenon, and has found information to both support and refute the concept by virtue of when such an occurrence could be expected.
“The consensus was it is a night time phenomena,” Woodfill said. “Apollo 13 entered in daylight in the Pacific and in Houston. Nevertheless, the question to this day demonstrates just how near Apollo 13 came to disaster. If the radio signal almost skipped off the Earth’s atmosphere, one wonders, just how very close was Apollo 13’s capsule and crew near to a fatal skipping into the oblivion of space as well.”
Another “angle” on Apollo 13’s reentry was how it very nearly escaped another potential disaster: landing in a typhoon.
“A tropical storm is a retro’s (retrofire officer) worst nightmare,” said Woodfill. “Knowing how unpredictable the movement and intensity of such storms are makes selecting a landing site difficult. No NASA reentry had ever landed in a tropical storm, and Apollo 13 might be the first. Among NASA scientists are meteorologists, and by their best science, they predicted that Tropical Storm Helen would move into the designated Apollo 13 landing site the day of reentry and splashdown.”
If Apollo 13 had splashed down amidst the storm, the capsule may have drifted and been lost at sea. To conserve the entry battery power, the beacon light recovery system had been deactivated. The crew would have been invisible to those looking for the capsule bobbing up and down in the Pacific Ocean. They eventually would have had to blow the hatch, and the Apollo 13 capsule likely would have sunk, similar to Gus Grissom’s Liberty Bell during the Mercury program. But the crew of Apollo 13 might not have been as fortunate as Grissom who had helicopter rescuers overhead quickly pulling him to safety.
However, the decision was made to ignore the weather forecasts, which ended up being fortuitous because Helen ultimately changed course. But then there was the uncertainty of the entry location due to the ‘shallowing’ the spacecraft was experiencing.
“Once more, the retro made the decision to ignore that shallowing at reentry in the same fashion as he had ignored the weathermen’s ominous prediction,” said Woodfill. “In both instances, the retro was correct. He rightly predicted that the drift would not be a problem in the final stages of reentry after the lander was jettisoned. Again, this was altogether fortuitous in that no one knew the lander’s cooling system was the source of the drift. Earlier, however, the retro had compensated for the shallowing drift by bringing Apollo 13 into the correct entry corridor angle via first having the crew fire the lander’s descent engine and later the lander’s thrusters.”
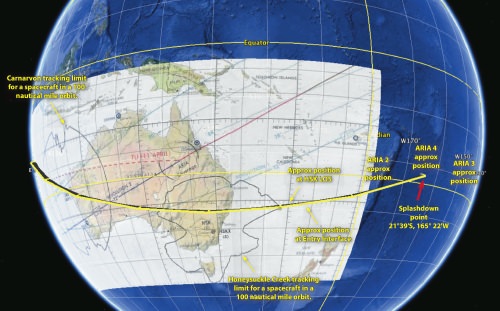
As it turned out those mysterious extra seconds caused by coming in at a shallow angle were also fortuitous.
While the added time of communications blackout was nail-biting, the more shallow and longer angle “added to the downrange path of Apollo 13, dropping the capsule in calm water so near the waiting aircraft carrier Iwo Jima that the accuracy was among the finest of the program,” Woodfill said.
Revisiting the length of the communications blackout, there are some discrepancies in various sources about the length of the extra time Apollo 13’s blackout time lasted. Some websites lists 25-30 seconds, others a minute. Again, I was unable to find an ‘official’ NASA statement on the subject and the transcript of the technical air to ground voice communications does not include time stamps for the beginning and end of blackout. Additionally, two of the definitive books about Apollo 13 – Lost Moon by Jim Lovell and Jeffrey Kluger, and A Man on the Moon by Andrew Chaikin – don’t give exact numbers on the timing of the blackout.
But Air & Space Magazine quoted Gene Kranz as saying it was 87 seconds.
“Per my mission log it started at 142:39 and ended at 142:45— a total of six minutes,” Kranz told journalist Joe Pappalardo in 2007. “Blackout was 1:27 longer than predicted … Toughest minute and a half we ever had.”
87 seconds also is confirmed by a transmission recorded on one of the ARIA, the Apollo/Advanced Range Instrumentation Aircraft, which provided tracking and telemetry information for the Apollo missions, especially at launch and reentry, when the Manned Spaceflight Network tracking could not.
ARIA 4 had the distinction of being the first to reacquire Apollo 13 after the longer-than-expected communication blackout, as it was near the predicted point of reentry. Captain David Dunn, who served as the Mission Coordinator onboard the ARIA 4 aircraft, provided a recording to historians at the Honeysuckle Creek Tracking Station, who have put together a wonderful history of their role in the Apollo missions.
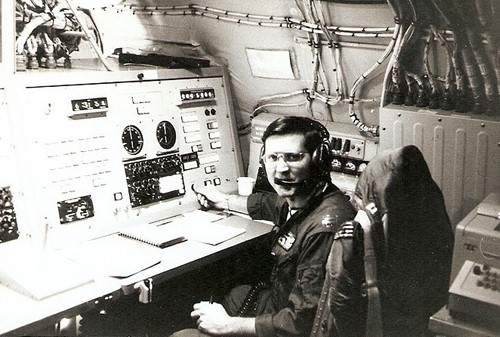
Space Historian Colin Mackellar from the Honeysuckle Creek website told Universe Today that until it was recently published on the Honeysuckle Creek website, the recording had not been heard by anyone other than Dunn’s family. Mackellar explained that it contains simultaneous audio of the NASA Public Affairs commentary, audio of the Flight Director’s loop, the ARIA transmissions and a portion of the Australian Broadcast Commission radio coverage.
Again, you can hear the palpable tension in the recording, which you can listen to at this link. At 7:21 in the audio, as communications blackout nears the predicted end, one of the ARIA communicators asks ARIA 4 if they can see the spacecraft. Negative is the reply.
At 7:55 you can hear Kranz asking if there is any acquisition of signal yet. Again at 8:43, Kranz asks, “Contact yet?” The answer is negative. Finally, at 8:53 in the audio, ARIA 4 reports AOS (acquisition of signal), which is relayed to Kranz. You can hear his relieved exhalation as he replies, “Rog (roger).”
Then comes Kranz saying, “Capcom, why don’t you try giving them a call.”
Capcom: “Odyssey, Houston standing by.”
Swigert: “OK, Joe.”
When the crew splashed down, the official duration time of the mission was 142 hours, 54 minutes and 41 seconds.
Dunn wrote about his experiences for the Honeysuckle Creek history website:
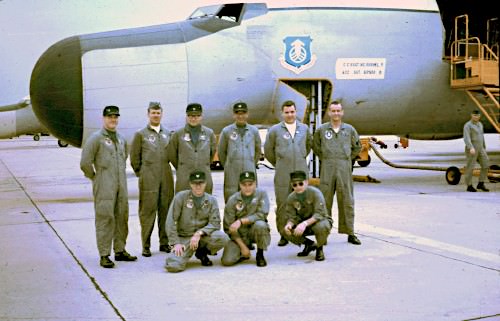
It required no great imagination to know that back in the US, and in fact all around the world, folks were glued to their TV sets in anticipation, and that Walter Cronkite was holding forth with Wally Schirra on CBS, and at the Houston Space Center breathing had ceased.
But we were there, ground zero, with front row seats and we would be the first to know and the first ones to tell the rest of the world if the Apollo 13 crew had survived…
On all the aircraft and all the airwaves there was complete silence as well as we all listened intently for any signal from Apollo 13.
ARIA 2 had no report of contact; ARIA 3 also had no report.
Then I observed a signal and Jack Homan, the voice radio operator advised me we had contact.
From Apollo 13 came the reply “OK, Joe……” relayed again from our radios to Houston and the rest of the world. Not much, but even such a terse reply was enough to let the world know the spacecraft and its crew had survived. In an age before satellite TV, teleconferencing, and the Internet, it was easy for us in the clouds at 30,000 feet above the splashdown zone to visualize breathing resuming in Houston and around the world.
Dunn concluded, “Now, exactly why would Ron Howard leave such a dramatic moment out of his film? There’s a real mystery!”
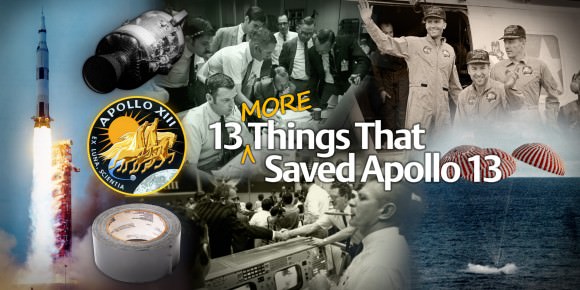
Tomorrow: Isolating the Surge Tanks
Previous articles in this series:
Part 1: The Failed Oxygen Quantity Sensor
Part 2: Simultaneous Presence of Kranz and Lunney at the Onset of the Rescue
Part 3: Detuning the Saturn V’s 3rd Stage Radio
Part 4: Early Entry into the Lander
Part 5: The CO2 Partial Pressure Sensor
Find all the original “13 Things That Saved Apollo 13″ (published in 2010) at this link.