We now know our stellar neighbors just a little better, and a new discovery may help tell us how common brown dwarfs are in our region of the galaxy. Early this week, researchers at Pennsylvania State University announced the discovery of a binary brown dwarf system. With a parallax measurement of just under 0.5”, this pair is only 6.5 light years distant making it the third closest system to our own and the closest example of the sub-stellar class of objects known as brown dwarfs yet discovered.
Named WISE J104915.57-531906, the system was identified by analysis of multi-epoch astrometry carried out by NASA’s Wide-field Infrared Survey Explorer (WISE). The discovery was made by associate professor of astronomy and astrophysics at Penn State’s Center for Exoplanets and Habitable Worlds Kevin Luhman. The system’s binary nature and follow up observations were confirmed by spectroscopic analysis carried out by the Gemini Observatory’s Multi-Object Spectrographs (GMOS).
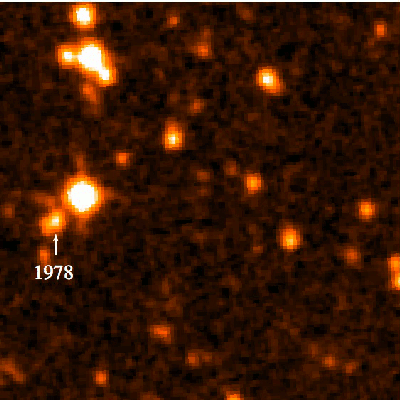
This find is also the closest stellar system discovered to our own solar system since the discovery of Barnard’s star by astronomer E.E. Barnard in 1916. Incidentally, Barnard’s star was the center of many spurious and controversial claims of extrasolar planet discoveries in the mid-20th century. Barnard’s star is 6 light years distant, and the closest star system to our own is Alpha Centauri measured to be 4.4 light years distant in 1839. In 1915, the Alpha Centauri system was determined to have a faint companion now known as Proxima Centauri at 4.2 light years distant. The Alpha Centauri system also made headlines last year with the discovery of the closest known exoplanet to Earth. WISE 1506+7027 is the closest brown dwarf to our solar system yet discovered. This also breaks the extended the All-WISE survey’s own previous record of the closest brown dwarf released in 2011, WISE 1506+7027 at 11.1 light years distant.
When looking for nearby stellar suspects, astronomers search for stars displaying a high proper motion across the sky. The very first parallax measurement of 11 light years distant was obtained by Friedrich Bessel for the star 61 Cygni in 1838. 61 Cygni was known as “Piazzi’s Flying Star” for its high 4.2” proper motion across the sky. To giving you an idea of just how tiny an arc second is, a Full Moon is about 1800” in diameter. With a proper motion of just under 3” per year, it would take WISE 1049-5319 over 600 years to cross the same apparent distance in the sky as viewed from the Earth!
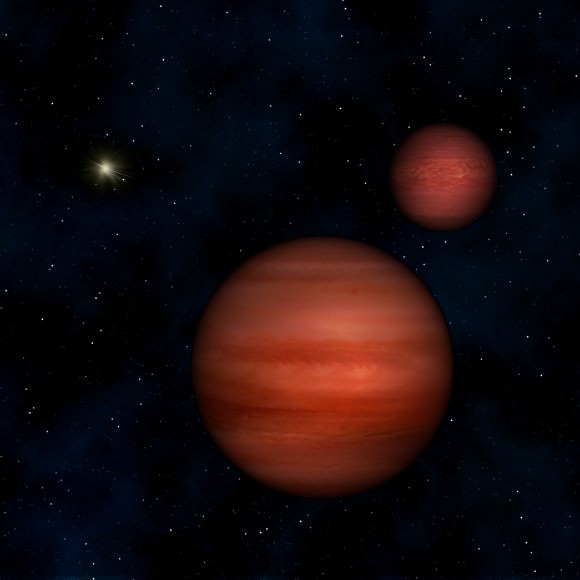
“Based on how this star system was moving in images from the WISE survey, I was able to extrapolate back in time to predict where it should have been located in older surveys,” stated Luhman. And sure enough, the brown dwarf was there in the Deep Near-Infrared Survey of the Southern Sky (DENIS), the Two Micron All-Sky Survey (2MASS) and the Sloan Digitized Sky Survey (SDSS) spanning a period from 1978 to 1999. Interestingly, Luhman also points out in the original paper that the pair’s close proximity to the star rich region of galactic plane in the constellation Vela deep in the southern hemisphere sky is most likely the reason why they were missed in previous surveys.
The discovery of the binary nature of the pair was also “an unexpected bonus,” Luhman said. “The sharp images from Gemini also revealed that the object actually was not just one, but a pair of brown dwarfs orbiting each other.” This find of a second brown dwarf companion will go a long way towards pinning down the mass of the objects. With an apparent separation of 1.5”, the physical separation of the pair is 3 astronomical units (1 AU= the Earth-Sun distance) in a 25 year orbit.
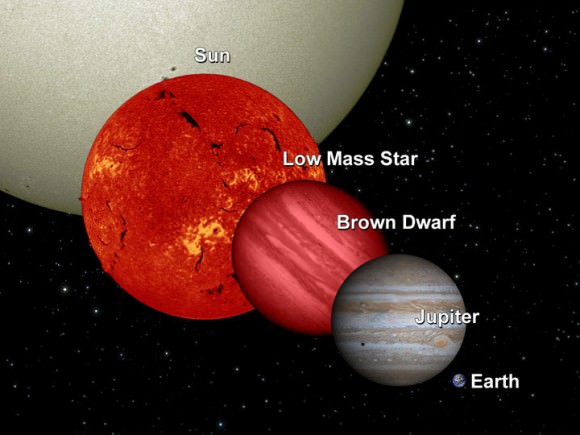
Brown dwarfs are sub-stellar objects with masses too low (below ~75 Jupiter masses) to sustain the traditional fusion of hydrogen into helium via the full proton-proton chain process. Instead, objects over 13 Jupiter masses begin the first portion of the process by generating heat via deuterium fusion. Brown dwarfs are thus only visible in the infrared, and run a spectral class of M (hottest), L, T, and Y (coolest). Interestingly, WISE 1049-5319 is suspected to be on the transition line between an L and T-class brown dwarf. To date, over 600 L-type brown dwarfs have been identified, primarily by the aforementioned SDSS, 2MASS & DENIS infrared surveys.
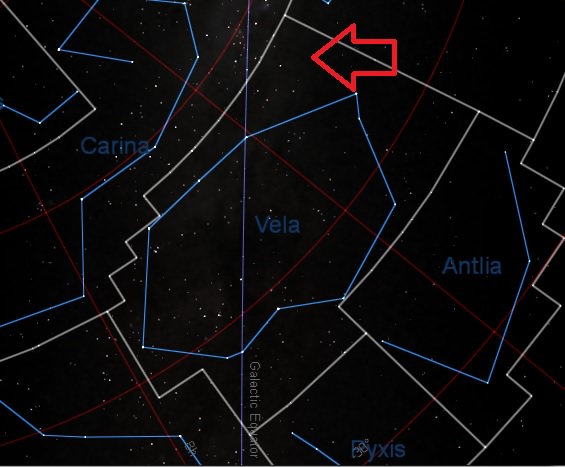
This discovery and others like it may go a long ways towards telling us how common brown dwarfs are in our region of the galaxy. Faint and hard to detect, we’re just now getting a sampling thanks to surveys such as WISE and 2MASS. The James Webb Space Telescope will do work in the infrared as well, possibly extending these results. Interestingly, Luhman notes in an interview with Universe Today that the potential still exists for the discovery of a brown dwarf closer to our solar system than Alpha Centauri. “No published study of the data from WISE or any other survey has ruled out this possibility… WISE is much more capable of doing this than any previous survey, but the necessary analysis would be fairly complex and time consuming. It’s easier to find something than to rule out its existence.” Said Luhman. Note that we’re talking a nearby brown dwarf that isn’t gravitationally bound to the Sun… this discussion is separate from such hypothetical solar companions as Nemesis and Tyche…and Nibiru conspiracy theorists need not apply!
The WISE 1049-5319 system is also a prime target in the search for nearby extra-solar planets. “Because brown dwarfs have very low masses, they exhibit larger reflex motions due to orbiting planets than more massive stars, and those larger reflex motions will be easier to detect.” Luhman told Universe Today. Said radial surveys for exoplanets would also be carried out in the IR band, and brown dwarfs also have the added bonus of not swamping out unseen planetary companions in the visible spectrum.
Congrats to Mr. Luhman and the Center for Exoplanets and Habitable Worlds on the discovery. You just never know what’s lying around in your own stellar backyard!
Read this original discovery paper here.