[/caption]
It’s a great question that’s now been validated by the Hubble Space Telescope. Recent observations have shown how galaxies are able to recycle huge amounts of hydrogen gas and heavy elements within themselves. In a process which begins at initial star formation and lasts for billions of years, galaxies renew their own energy sources.
Thanks to the HST’s Cosmic Origins Spectrograph (COS), scientists have now been able to investigate the Milky Way’s halo region along with forty other galaxies. The combined data includes instruments from large ground-based telescopes in Hawaii, Arizona and Chile whose goal was determine galaxy properties. In this colorful instance, the shape and spectra of each individual galaxy would appear to be influenced by gas flow through the halo in a type of “gas-recycling phenomenon”. The results are being published in three papers in the November 18 issue of Science magazine. The leaders of the three studies are Nicolas Lehner of the University of Notre Dame in South Bend, Ind.; Jason Tumlinson of the Space Telescope Science Institute in Baltimore, Md.; and Todd Tripp of the University of Massachusetts at Amherst.
The focus of the research centered on distant stars whose spectra illuminated influxing gas clouds as they pass through the galactic halo. This is the basis of continual star formation, where huge pockets of hydrogen contain enough fuel to ignite a hundred million stars. But not all of this gas is just “there”. A substantial portion is recycled by both novae and supernovae events – as well as star formation itself. It not only creates, but “replenishes”.
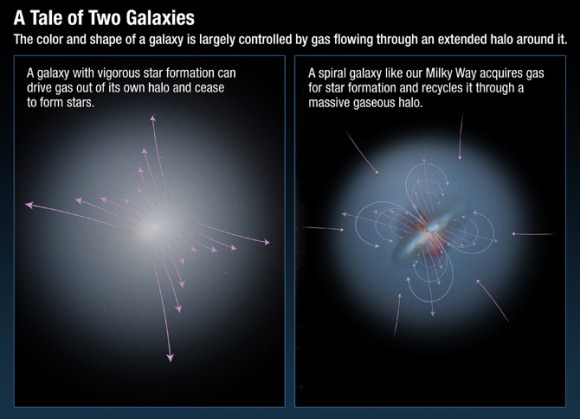
However, this process isn’t unique to the Milky Way. Hubble’s COS observations have recorded these recycling halos around energetic star-forming galaxies, too. These heavy metal halos are reaching out to distances of up to 450,000 light years outside the visible portions of their galactic disks. To capture such far-reaching evidence of galactic recycling wasn’t an expected result. According to the Hubble Press Release, COS measured 10 million solar masses of oxygen in a galaxy’s halo, which corresponds to about one billion solar masses of gas – as much as in the entire space between stars in a galaxy’s disk.
So what did the research find and how was it done? In galaxies with rapid star formation, the gases are expelled outward at speed of up to two million miles per hour – fast enough to be ejected to the point of no return – and with it goes mass. This confirms the theories of how a spiral galaxy could eventually evolve into an elliptical. Since the light from this hot plasma isn’t within the visible spectrum, the COS used quasars to reveal the spectral properties of the halo gases. Its extremely sensitive equipment was able to detect the presence of heavy elements, such as nitrogen, oxygen, and neon – indicators of mass of a galaxy’s halo.
So what happens when a galaxy isn’t “green”? According to these new observations, galaxies which have ceased star formation no longer have gas. Apparently, once the recycling process stops, stars will only continue to form for as long as they have fuel. And once it’s gone?
It’s gone forever…
Original Story Source: Hubble Space Telescope News Release.