[/caption]
Former NASA astronaut Jim Lovell came under fire last week when he sold a personal memento from his tenure with the space agency at an auction – the 70-page checklist from the famous Apollo 13 mission that didn’t land on the Moon. The sale has reopened the ongoing debate over who owns NASA artifacts and photographs, the astronauts or the public.
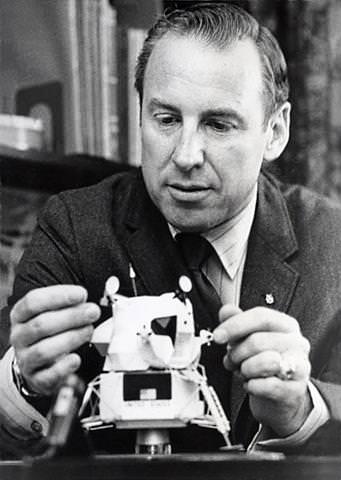
In Lovell’s case, the checklist is so valuable because it contains Lovell’s hand written calculations he used to navigate the crippled Apollo 13 spacecraft after its oxygen tank exploded. That’s a pretty important piece of history for many collectors. Bids on the historic item surpassed $388,000.
But now NASA is questioning whether Lovell had the right to sell the item and profit from its sale. For now, the checklist – along with a lunar module identification plate and a hand controller from Apollo 9 sold by former astronaut Rusty Schweickart and a glove Al Shepard wore on the Moon on Apollo 14 sold at the same auction – is locked in a Heritage Auctions vault until the issue is resolved.
NASA administrator Charles Bolden released a statement saying that there have been “fundamental misunderstandings and unclear policies” regarding items astronauts took home from the Mercury, Gemini, Apollo and Skylab mission.
These “misunderstandings and unclear policies” aren’t new. Last summer, NASA filed a lawsuit against Apollo 14 astronaut Ed Mitchell after he tried to sell a 16mm video camera he used on the Moon. NASA claimed Mitchell was selling the camera illegally and sued the former astronaut for ownership rights. Mitchell countered that the camera would have been left on the Moon had he not brought it home. It’s been sitting in his personal safe since 1971.
Mitchell isn’t wrong in his self defense. In the 1960s and 1970s, NASA officials told the astronauts that they could keep certain equipment from the missions.
In 2002, former Flight Director Chris Kraft said that he approved the policy. Apollo astronauts were allowed to keep personal items that flew with them as well anything from the lunar landing module that would otherwise have been abandoned on the Moon. The astronaut had great freedom in choosing what they wanted to keep.
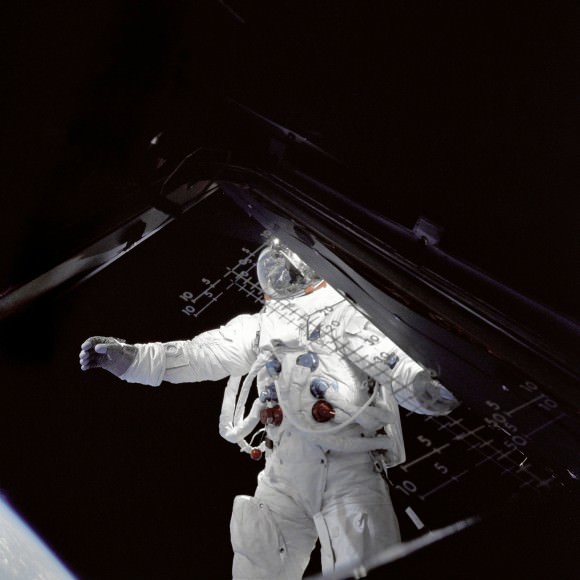
“It was generally accepted that the astronauts could bring back pieces of equipment or hardware from this spacecraft for a keepsake of these journeys,” Kraft wrote.
Since the end of the space race, collectors around the world have paid millions to own pieces of history themselves. NASA’s problem isn’t with these former astronauts keeping pieces of history for themselves, it’s when they sell these artifacts for personal gain that creates a problem.
Kraft’s 2002 letter doesn’t address whether or not astronauts have the right to sell their mementos. In its recent letter to the auction house, NASA insisted only the agency can approve such artifacts for sale.
Bolden said the ownership discussions will explore “all policy, legislative and other legal means” to resolve ownership issues “and ensure that appropriate artifacts are preserved and available for display to the American people.” The agency has agreed to work cooperatively with the astronauts to resolve what’s recently become a contentious issue.
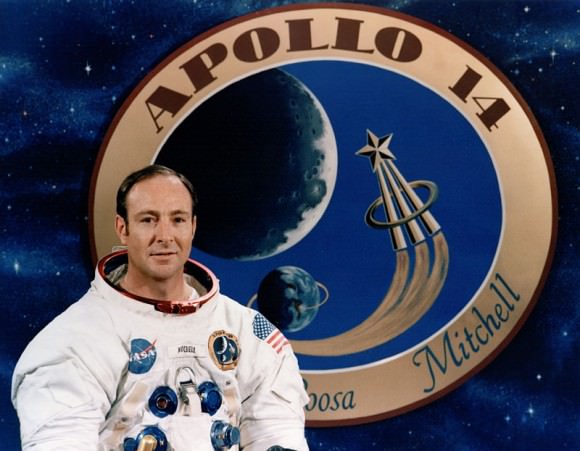
It is a bit of a grey area. The astronauts did the work, they trained for difficult mission and went to the Moon. But NASA footed the bill, and American tax payers funded NASA. The space agency argues that artifacts from the Apollo era should be available to the public. Everyone should be able to view and experience these pieces of one of the nation’s historic achievements.
Source: Yahoo! News