Weekend SkyWatcher’s Forecast: February 19-21, 2010
Greetings, fellow SkyWatchers! It’s a lunatic weekend as we head towards the Moon’s surface to study its features. Need more of a challenge? Then we’ll take a look at an area of sky where a Sakurai’s Object may have appeared. Just into stargazing? Then watch the Pleiades and the Moon dance together as our weekend ends! Whenever you’re ready, I’ll see you in the backyard…
February 19, 2010 – On this date we celebrate the 1473 birth of Nicolaus Copernicus, creator of the modern Solar System model.
‘‘Finally we shall place the Sun himself at the center of the Universe. All this is suggested by the systematic procession of events and the harmony of the whole Universe, if only we face the facts, as they say, ‘with both eyes open.’’’
Take a look at Copernican theory just as the master did:
‘‘For a traveler going from any place toward the north, that pole of the daily rotation gradually climbs higher, while the opposite pole drops down an equal amount… I shall now recall to mind that the motion of the heavenly bodies is circular, since the motion appropriate to a sphere is rotation in a circle.’’
Tonight we’ll explore a heavenly body – the Moon – as we take out binoculars or a telescope to explore “The Sea of Nectar”…
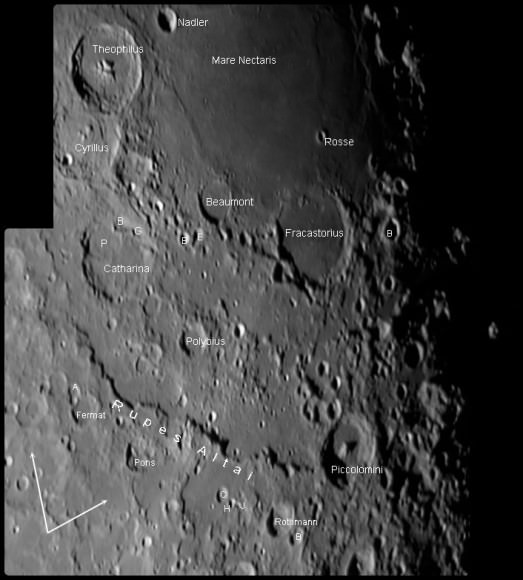
At around 1000 meters deep, Mare Nectaris covers an area of the Moon equal to that of the Great Sandhills in Saskatchewan, Canada. Like all maria, it is part of a gigantic basin which is filled with lava, and there is evidence of grabens along the western edge of the basin. While Nectaris’ basaltic flows appear darker than those in most maria, it is one of the older formations on the Moon, and as the terminator progresses you’ll be able to see where ejecta belonging to Tycho crosses its surface. For a real challenge, look for an ancient and ruined crater which lies on the southern shore of Mare Nectaris. To binoculars, Fracastorius will look like a shallow, light colored ring, but a telescope will reveal its northern wall is missing – perhaps melted away by the lava flow which formed the mare. This is all that remains of a once grand crater which was more than 117 kilometers in diameter. The tallest of its eroded walls still stand at an impressive 1758 meters, placing them as high as the base elevation of Mt. Hood, yet in places nothing more than a few ridges and low hills still stand to mark the crater’s remains. Power up and look for interior craterlets. Be sure to mark your lunar observing challenge notes with your observations! As Copernicus would have said:
‘‘Although all the good arts serve to draw man’s mind away from vices and lead it toward better things, this function can be more fully performed by this art, which also provides extraordinary intellectual pleasure.’’
February 20, 2010 – On this date in 1962, John Glenn was rocketing around Earth on his first orbit as our friends ‘‘down under’’ made history. Residents of Perth, Australia, simultaneously switched on lights as Glenn flew over—the first city spotted from space! When you’re ready, let’s change our perception of the size of the things we see on the lunar surface by exploring the edges of Mare Serenitatus – a feature that’s about the same size as the state of New Mexico.

On its southwest border stands the Haemus Mountains, which will continue on beyond the terminator. Look in their midst for the sharp punctuation of Class I Menelaus. This small crater has a brilliant west inner wall and deeply shadowed floor. Like Taruntius, Menelaus is another fine crater to watch for expansive ray systems as the terminator progresses. While the Montes Haemus look pretty impressive, they are nothing more than foothills compared to the Apennines which have yet to emerge into the sunlight. Look at Serenitatus’ northwest edge to view some real mountains! These are the Montes Caucasus, rising up to 5182 meters above the plains. Look closely at the maps and you will find this is also home to the Apollo 11, Apollo 16 and Apollo 17 landers, as well as Luna 21. It is an area that you can deeply appreciate for its historical significance. Like its earthly counterpart, the Caucasus Mountain Range has peaks that reach upwards of six kilometers – summits as high as Mount Elbrus! Nearby and slightly smaller than its terrestrial namesake, the lunar Apennine mountain range extends some 600 kilometers with peaks rising as high as five kilometers. Be sure to look for the summit of Mons Hadley, one of the tallest peaks you will see at the northern end of this chain. It rises above the surface to a height of 4.6 kilometers, making that single mountain about the size of asteroid Toutatis.
Today in 1996 also marks the discovery of Sakurai’s Object, a star in collapse.While studying Sagittarius and photographing what appeared to be a typical nova, Yukio Sakurai became only the third twentieth-century astronomer to witness a star in final helium flash. When this occurs, the star is switching its nuclear fuel from hydrogen to helium and then burning the helium to carbon in the final stage, burping forth an envelope from its interior.
If you’re up to a challenge, then wait until the Moon is set and let’s examine an open cluster where stars have gone through this same evolutionary step. Begin by identifying Delta Geminorum and hop a fist-width east for open cluster NGC 2420 (RA 07 38 23 Dec +21 34 24). This magnitude 8.5 group is visible under dark-sky conditions to binoculars as a weak, round, hazy patch and requires a mid-sized telescope to begin resolution of its long, looping chains of stars. Some members are similar to Sakurai’s Object, while others have evolved to helium depletion. Studying clusters like NGC 2420 is important: they are areas where stars are all about the same age, yet their different masses mean they evolve at different rates. Average telescopes will only see the primary stars, while large aperture notices the distinct glow of hundreds of stars on the verge of resolution. If you get the impression of a weak globular cluster, you’d be correct. With a thousand members packed into a 30-light-year sphere, a lot has happened during its 1.7-billion-year lifetime. It may have started in our own galaxy’s cluster-forming region and been thrown clear by an encounter with a large mass. Or, it might have once been part of a smaller galaxy absorbed by our own. But one thing is clear: its unusual Sun-like elements so far from where they belong make NGC 2420 a prime playground for study. Some of its members could even be blue stragglers—unions of two stars into one!
February 21, 2010 – This day in 1972, Luna 20 made a safe touchdown in the Apollonius highlands, where it captured 30 grams of surface material to return to Earth. Now it’s time to look take a closer look at Sinus Medii – the “Bay in the Middle” of the visible lunar surface.
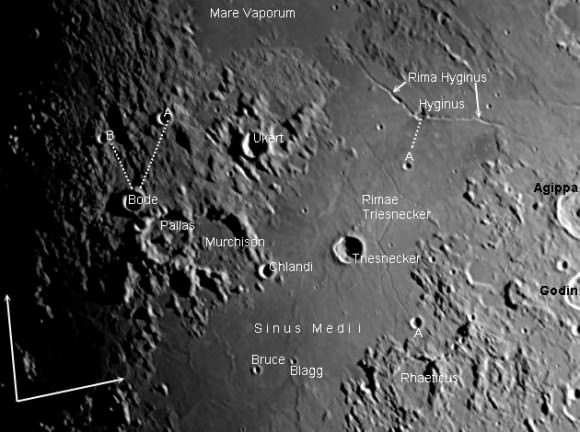
Central on the terminator, and the adopted “center” of the lunar disc, this the point from which latitude and longitude are measured. This smooth plain may look small, but it covers about as much area as the states of Massachusetts and Connecticut combined. During full daylight temperatures in Sinus Medii can reach up to 212 degrees! On a curious note, in 1930 Sinus Medii was chosen by Edison Petitt and Seth Nicholson for a surface temperature measurement at full Moon. Experiments of this type were started by Lord Rosse as early as 1868, but on this occasion Petit and Nicholson found the surface to be slightly warmer than boiling water. Around a hundred years after Rosse’s attempt, Surveyor 6 successfully landed in Sinus Medii on November 9, 1967, and became the very first probe to “lift off” from the lunar surface.
Now, just kick back and relax as you take a closer look at what’s around the Moon to discover the Pleiades! For a great deal of western Africa, this is an occultation event – so be sure to check IOTA for times and information in your area!
Until next week? Dreams really do come true when you keep on reaching for the stars!
This week’s awesome lunar shots were done by Peter Lloyd and Greg Konkel. NGC 2420 – Credit: Palomar Observatory, courtesy of Cal Tech.