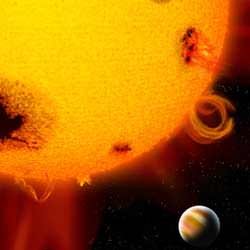
Artist illustration of a planet orbiting a very young, active star. Image credit: UFL. Click to enlarge.
Astronomers have discovered a planet orbiting a very young star nearly 100 light years away using a relatively small, publicly accessible telescope turbocharged with a new planet-finding instrument.
The feat suggests that astronomers have found a way to dramatically accelerate the pace of the hunt for planets outside our solar system.
“In the last two decades, astronomers have searched about 3,000 stars for new planets,” said Jian Ge, a professor of astronomy at the University of Florida. “Our success with this new instrument shows that we will soon be able to search stars much more quickly and cheaply ? perhaps as many as a couple of hundred thousand stars in the next two decades.”
Ge and colleagues at the University of Florida, Tennessee State University, the Institute of Astrophysics in Spain’s Canary Islands, Pennsylvania State University and the University of Texas presented their findings today at the American Astronomical Society’s annual meeting in Washington, D.C.
Their work is important in part because of what the astronomers found ? a planet, at least half as massive as Jupiter, orbiting a star just 600 million years old. That’s very young compared, for example, with the sun’s 5 billion years.
“This is one of the youngest stars ever identified with a planetary companion,” Ge said. Perhaps more significant, the instrument used to find the planet points the way to a much more accessible method for finding others ? including those capable of supporting life.
Planets outside our solar system are typically swamped by the light of their stars, making it difficult to observe them visually. In the 1990s, astronomers began using a measurement technique called Doppler radial velocity to detect planets by observing the wobble in a star that is gravitationally induced by an orbiting planet.
This technique, which has uncovered the vast majority of the 160-plus extrasolar planets found so far, works by hunting through the spectrum of starlight for the subtle Doppler shifts that occur as the star and planet move toward and away from their common center of mass. The instrument at the heart of this technique is usually a spectrograph, but this instrument is problematic.
“A major problem with spectrographs is that they collect only a small percentage of photons from the target light source, which means that they are only useful to search for distant planets when mounted on relatively large telescopes,” Ge said.
The astronomers’ new instrument, the Exoplanet Tracker, or ET, eliminates this problem by swapping the spectrograph with an interferometer, a device that can take more precise radial velocity measurements. Tests show the interferometer can capture as much as 20 percent of available photons, making the instrument far more powerful, which opens its use for distant planet hunting to smaller telescopes.
At a development cost of about $200,000, the interferometer-equipped ET is also far cheaper than comparable spectrographs, which cost more than $1 million. And at about 4 feet long, 2 feet wide and weighing about 150 pounds, it is lighter and smaller. The instrument is based on a concept first proposed in 1997 by Lawrence Livermore National Lab physicist David Erskine.
The astronomers used the Exoplanet Tracker on the special 0.9-meter Coud? feed system within the National Science Foundation’s 2.1-meter telescope at Kitt Peak National Observatory near Tucson, Ariz.
Like radial velocity instruments equipped with spectrographs, the ET instrument in its present form can search only one object at a time. But Ge’s team has demonstrated that it can hunt for planets around multiple stars simultaneously ? a key element of its heightened utility. The team is working on a version capable of surveying as many as 100 stars simultaneously.
The Exoplanet Tracker will be used next spring for a trial planet survey on the Sloan Digital Sky Survey 2.5 meter wide-field telescope at the Apache Point Observatory in New Mexico. The new instrument is funded with an $875,000 grant from the W.M. Keck Foundation. A much more ambitious, long-term survey is in the planning stages.
The Kitt Peak Coud? feed telescope that Ge and colleagues used to discover the new planet has a 0.9-meter mirror on a tall tower, a mirror that directs incoming starlight into an observing room in the base of the 2.1-meter telescope. The standard spectrograph in the facility fills the room ? while ET occupies a small corner.
The new planet is the most distant ever found using the Doppler technique with a telescope mirror less than 1 meter in size. There are hundreds of such telescopes worldwide, compared with just a handful of the larger 2- and 3-meter telescopes more commonly used in planet finding ? telescopes that tend to be in extremely high demand and difficult to access.
“These smaller telescopes are relatively cheap and relatively available,” Ge said, “so you can often get access to many dozens of nights on them if you have a promising proposal.”
Kitt Peak National Observatory is part of the National Optical Astronomy Observatory, Tucson, Ariz., which is operated by the Association of Universities for Research in Astronomy Inc., under a cooperative agreement with the National Science Foundation.
“This is the first time that a planet has been discovered using a publicly funded telescope at the U.S. national observatory,” said Buell Jannuzi, acting director of Kitt Peak National Observatory. “We are very excited that the broader community of astronomers around the world will be able to propose to use the single-object Exoplanet Tracker instrument at Kitt Peak to carry out their own research programs, starting in the fall of 2006.”
That said, discovering new planets is never easy.
In the latest find, astronomers went to great lengths to ensure they were actually “seeing” a planet. That’s because the star, which has about 80 percent of the mass of our sun, retains much of its youthful rotation speed, which makes it capable of generating strong magnetic fields and associated dark star spots. These are similar to the magnetically generated sunspots on our own sun, and they can mimic the presence of a planet in orbit around the star.
To check against this possibility, Greg Henry, an astronomer at Tennessee State, observed the star with an automated telescope in Arizona, and found the star to be changing its brightness as it rotates.
“My observations reveal a rotation period of about 12 days for the star,” Henry said. “Thus, if the planetary orbital period is indeed less than five days, the dark spots rotating around on the surface of the star every 12 days cannot be causing the false appearance of a planet.”
Located in the direction of the constellation Virgo, the newly discovered planet completes its orbit in less than five days, meaning it orbits very close to its parent star and is very hot. That means it’s too close to the star to lie within the “habitable zone” where life is possible.
Original Source: UFL News Release