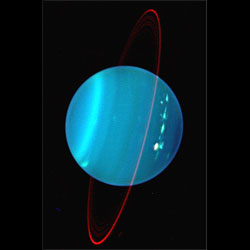
Uranus and its faint ring system which was discovered this week in 1977. Image credit: NASA/JPL. Click to enlarge.
If you haven’t had the chance to catch bright comet Pojmanski, the time is now. Head out this week to catch lunar features, bright stars and open clusters. Keep on looking because…
Here’s what’s up!
Monday, March 6 – Still making headlines, bright comet 2006/A1 Pojmanski is on the move. Easily spotted well before dawn with even small binoculars, Pojmanski has a very bright nucleus accompanied by a large, green-hued coma – along with reports of up to two degrees of tail. Now cruising through Aquila at an average magnitude of 5.4, it will continue to fade until it reaches Lacerta at month’s end. SkyHound provides excellent locator charts. Take the opportunity to locate this fine comet before the Moon returns to morning skies!
If you see sunshine today, celebrate the birthday of Joseph Fraunhofer born this date in 1787. The German scientist Fraunhofer was a true “trailblazer” of modern astronomy and his field was spectroscopy. After having served an apprenticeship as a lens and mirror maker, Fraunhofer went on to develop specialized optical instruments. While designing the modern achromatic objective lens for the telescope, he watched the sun’s light passing through a thin slit and saw many dark lines – part of the “rainbow bar code.” Fraunhofer knew some of these lines could be used as a wavelength “standard.” For this reason he began measuring their locations relative to one another. The most prominent of the lines he labeled with letters still in use today. His skill in optics, mathematics, and physics led Fraunhofer to design and build the very first diffraction grating capable of measuring the wavelengths of specific colors and dark lines in the solar spectrum. And his telescope – did it succeed? Of course. The achromatic objective lens is still a design of choice, and the binoculars you have? They’re achromats!
Tonight will be the perfect opportunity to find the lunar crater named for Fraunhofer. Return again to the now shallow appearing crater Furnerius. Can you spot the ring at its southern edge? This is crater Fraunhofer – a challenge under these lighting conditions.
Now, revisit the “Twin Stars” – Castor and Pollux. Separated by not much more than 3 arc seconds, 2.0 magnitude Castor A has a bright sibling – 2.8 magnitude Castor B. The pair is actually a true binary with an orbital period of roughly 500 years. The Castor system contains four lesser members – each main star is a spectroscopic binary. Without Fraunhofer’s discovery, we would have never known.
Although spectroscopes and telescopes are powerful instruments able to reveal much, sometimes you just have to get close for more details. Today in 1986, the first of over a week of flybys began as the Russian built VEGA 1 and European Space Agency’s Giotto became the first space probes to reach Halley’s Comet.
Tuesday, March 7 – On this date in 1792, the only child of William Herschel was born – John. Herschel became the first astronomer to thoroughly survey the southern hemisphere sky and he discovered photographic fixer – an important chemical ingredient needed to preserve images on photographic plates. Also born on this date in 1837 was Henry Draper, the man who made the first photograph of Vega’s stellar spectrum in 1872. Eight years later, he took the first picture of the Great Nebula in Orion. Draper’s contribution led to new techniques in astrophotography, making it possible for celluloid to reveal faint detail beyond the reach of the eye in the 1880’s. This led to development of the Great Observatories – and telescopes – necessary to ultimately show an expanding cosmos populated by numberless “island universes” beyond our own Milky Way.
Tonight’s outstanding lunar features are two craters that you simply can’t miss – Aristotle and Eudoxus. Located to the north, this pair will be highly prominent in binoculars as well as telescopes. The northernmost – Aristotle – was named for the great philosopher and has an expanse of 87 kilometers. Its deep, rugged walls show a wealth of detail at high power, including two small interior peaks. Companion crater Eudoxus, to the south, spans 67 kilometers and offers equally rugged detail.
If you haven’t been following Saturn with regularity, tonight’s bright Moon might make this an occasion to spend some quality time on the ring system and satellites. At magnifications above 100x, the main division separating Ring A from B (Cassini’s Division) should be readily apparent in most scopes. Try making a series of simple sketches showing any nearby “stars.” Keep the sketch as the Moon waxes to full and see if you can distinguish between background stars and the planet’s own retinue of six most easily observed satellites – Titan, Rhea, Tethys, Dione, Enceladus, and Iapetus.
Wednesday, March 8 – On this day in 1977, NASA’s airborne occultation observatory made an unexpected discovery – Uranus had rings. Human eyes didn’t actually see Uranus’ faint ring system at the time – only the strange wink of a star hidden behind them. Imaging the rings had to wait until Voyager 2 whisked by nine years later.
Tonight the Moon provides a piece of scenic history as we take a more in-depth look at a previous study crater – Albategnius. This huge, hexagonal, mountain-walled plain appears near the terminator about one-third the way north of the south limb. This 135 kilometer wide crater is approximately 14,400 feet deep and its west wall casts a black shadow on the dark floor. Partially filled with lava after creation, Albategnius is a very ancient formation that later became home to several wall-breech craters, such as Klein, which can be seen telescopically on the southwest wall. Albategnius holds more than just the distinction of being a prominent crater tonight – it also holds a place in history. On May 9, 1962 Louis Smullin and Giorgio Fiocco of the Massachusetts Institute of Technology (MIT) aimed a ruby laser beam toward the Moon’s surface and Albategnius became the first lunar feature to reflect laser light from Earth.
On March 24, 1965 Ranger 9 took a “snapshot” of Albategnius from an altitude of approximately 2500 km. Ranger 9 was designed by NASA for one purpose – to achieve lunar impact trajectory and send back high-resolution photographs and video images of the lunar surface. Ranger 9 carried no other science packages. Its destiny was to simply take pictures right up to the moment of impact. They called it… a “hard landing.”
Thursday, March 9 – Today is the 442nd anniversary of David Fabricius’ birth. Born in 1564, Fabricius discovered the first variable star – Mira. At the heart of Cetus the Whale, it is now dipping steeply to the south-southwest at skydark. Even when well placed above the horizon, you can’t always count on Mira being seen. At its brightest, Mira achieves magnitude 2.0 – bright enough to be seen 10 degrees above the horizon. However Mira “the Wonderful” can also get as faint as magnitude 9 during its 331 day long “heartbeat” cycle of expansion and contraction. Mira is regarded as a premiere study for amateur astronomers interested in beginning variable star observations. For more information about this fascinating and scientifically useful branch of amateur astronomy contact the AAVSO (American Association of Variable Star Observers).
Tonight’s featured lunar crater is located on the south shore of Mare Imbrium right where the Apennine mountain range meets the terminator. At 58 kilometers in diameter and 12,300 feet deep, Eratosthenes is an unmistakable crater. Named after the ancient Greek mathematician, geographer and astronomer Eratosthenes, this splendid crater will display a bright west wall and a black interior hiding its massive crater capped central mountain 3570 meters high! Extending like a tail, an 80 kilometer mountain ridge angles away to its southwest. As beautiful as Eratosthenes appears tonight, it will fade away to almost total obscurity as the Moon approaches full. See if you can spot it again in five days.
Friday, March 10 – Tonight would be a terrific opportunity to study under-rated crater Bullialdus. Located close to the center of Mare Nubium, even binoculars can make out Bullialdus when near the terminator. If you’re scoping – power up – this one is fun! Very similar to Copernicus, Bullialdus’ has thick, terraced walls and a central peak. If you examine the area around it carefully, you can note it is a much newer crater than shallow Lubiniezsky to the north and almost non-existent Kies to the south. On Bullialdus’ southern flank, it’s easy to make out its A and B craterlets, as well as the interesting little Koenig to the southwest.
Despite the bright waxing moon, we still have a chance to get a view of a sprinkling of faint stars high to the south at skydark. Located less than a finger-width west-northwest of Wezen (Delta Canis Majoris) – 6.5 magnitude NGC 2354 is achievable in small scopes. Although richly populated, this open cluster lacks a bright core. This may challenge the eye to see it. Despite the moonlight, about a dozen stars should be visible in smaller scopes, but return on a moonless night to look for faint clumps and chaining among its 50 or so brightest members.
Saturday, March 11 – Today celebrates the birth of Urbain Leverrier. Born in 1811, Leverrier predicted the existence of Neptune. Along with a similar prediction by John Couch Adams, this led to its discovery. As both a mathematician and astronomer, Leverrier was also the first scientist to promote the idea of daily weather forecasts.
Tonight we’ll have the opportunity to look for a lunar feature named for Leverrier. To find it, start with the C-shape of Sinus Iridum. Imagine that Iridum is a mirror focusing light – this will lead your eye to crater Helicon. The slightly smaller crater southeast of Helicon is Leverrier. Be sure to power up to capture the splendid north-south oriented ridge which flows lunar east.
Tonight let’s try a lovely triple star system – Beta Monocerotis. Located about a fist width northwest of Sirius, Beta is a distinctive white star with blue companions. Separated by about 7 arc seconds, almost any magnification will distinguish Beta’s 4.7 magnitude primary from its 5.2 magnitude secondary to the southeast. Now, add a little power and you’ll see the fainter secondary has its own 6.2 magnitude companion less than 3 arc seconds away to the east.
Sunday, March 12 – Tonight let’s turn binoculars or telescopes toward the southern lunar surface as we set out to view one of the most unusually formed craters – Schiller. Located near the lunar limb, Schiller appears as a strange gash bordered on the southwest in white and black on the northeast. This oblong depression might be the fusion of two or three craters, yet shows no evidence of crater walls on its smooth floor. Schiller’s formation still remains a mystery. Be sure to look for a slight ridge running along the spine of the crater to the north through the telescope. Larger scopes should resolve this feature into a series of tiny dots.
Want a challenging double this evening? Then let’s have a look at Theta Aurigae. 2.7 magnitude Theta is a four star system ranging in magnitudes from 2.7 to 10.7. The brightest companion – Theta B – is magnitude 7.2 and is separated from the primary by slightly more than 3 arc seconds. Remember that this is what is known as a “disparate double” and look for the two fainter members well away from the primary.
Grab a comet by the tail and may all your journeys be at light speed! …~Tammy Plotner. Contributing author: Jeff Barbour @ astro.geekjoy.com