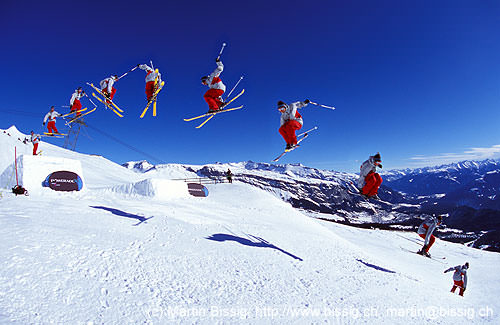
“Go big or go home.” That’s what aerialists on the US Olympic ski team say, and when they say “big,” they mean it.
Big means “Big Air,” 20 meters above the ground, as high as a five-story building. Aerial skiers fly into the void as fast as a motorcycle speeds down a city street, flipping head over heels, twisting and flipping again. The sky tumbles, but dizziness is not allowed, because only 3 heart-pumping seconds after launch, it’s time to land.
“And you don’t want to land on your ? well ? you know,” says aerial skier and Olympic gold medalist Eric Bergoust: educational video.
He should know. In the sport of aerial skiing, Bergoust has done it all. He was one of the first skiers ever to complete a quad-twist triple flip–four twists and three flips in mid-air. In 1998, hours after a frightening crash in practice, he used the move to win gold at the Nagano Olympic Games. At the time, his score was the highest ever recorded. This year, he’s a top contender again in Torino.
Bergoust has a knack for invention. He has designed new skis to soften the impact of practice landings in swimming pools. He has altered the shape of ski jumps, called “kickers,” to make flights longer-lasting and safer. And his take-off method, raising one arm propeller-style to add twist to his flight, is widely imitated.
His next innovation: “We should jump on the moon! There’s plenty of fresh powder (moondust),” he explains. “And I figure the 1/6 g would give us a lot of hang time.” More hang time means more flips–and more gold.
Consider the following:
On Earth, a typical run begins with Bergoust hurtling down a 23-degree slope. By the time he reaches bottom, 20 meters below the starting gate, he’s traveling almost 70 km/hr?directly into the kicker. From a skier’s point of view, the kicker looks uncomfortably like a wall, but it’s really a ramp guiding the aerialist almost straight up in the air. Bergoust’s favorite kickers are angled at 70 degrees! Up he goes, hanging for nearly 3 seconds before landing in soft snow another 20+ meters beyond the ramp.
see captionNow imagine the same run?same hill, same kicker, same skier?on the moon. Because lunar gravity is less, Bergoust would accelerate downslope at a more gradual pace, reaching bottom with a speed of only 28 km/hr. On Earth, such a slow start would be a disaster. On the moon, it’s perfect. Leaving the kicker at that speed, Bergoust hangs in the “air” for a whopping seven seconds, more than twice his hang-time on Earth: proof.
“I might be able to double my quad-triple,” he says.
Remember, Bergoust won gold in 1998 with a quad-triple. Since then other skiers have added a single twist to his move, turning it into a quint-triple. “Quints” are expected to win the men’s freestyle aerials in Torino. On the moon, Bergoust would have time to add four more twists and three more flips to his routine. “Let’s see?” calculates Bergoust, “that would be an octuple-twist sextuple flip.” Guaranteed gold.
Now for the problems:
skiing on the moonMoondust, although it is powdery, is not as slippery as snow. On the contrary, moondust is very abrasive. It is made of tiny sharp fragments of glass and rock produced by eons of meteoroids pulverizing the moon. Compared to snow, moondust is a “slow surface,” maybe too slow for a good jump.
To combat this, skiers are going to need extra-slick skis coated with Teflon or some other low-friction material. Thin films of diamond might be the answer. Diamond-like carbon films in Earth laboratories rival Teflon in slipperiness, with the advantage of diamond-like hardness to resist the scratching action of sharp-edged dust.
Another problem is the kicker. On Earth, kickers are made of snow. Workers blow snow into large wooden forms laid out at the base of the slope. A spray of water helps the snow stick together and makes the ramp slippery-smooth. Disassemble the forms and?voila!?a kicker.
Imagine the same process on the moon. Workers assemble their form and begin dumping moondust into it. There’s no water hose to squirt the dust to make it stick together. Water exposed to lunar vacuum sublimates (vaporizes) in a flash. So the dust remains dry. Disassemble the forms and?voila!?the kicker slumps into a shapeless pile.
The solution in this case might be a microwave water hose. In labs on Earth, researchers have discovered that grains of moondust cooked in a microwave oven quickly melt and stick together. Spraying moondust with microwaves might allow Olympic workers to mold a good kicker.
And finally?the landing.
On Earth, aerial skiers land on a layer of soft snow, which cushions their impact. On the moon, they’ll land on a layer of soft moondust. Very likely, the dust will spray upwards, coating the skier’s suit.
What’s the problem? Ask any Apollo astronaut. They hated it when moondust got on their spacesuits. Dark dust absorbed sunlight, causing the suit to overheat. Sharp edges of the dust cut into seals, springing leaks. Dust-covered visors were hard to see through. A skier’s suit, thoroughly “dusted,” might be useless after a single run. Another problem to solve….
Bergoust loves solving problems. For years he’s been tinkering with skis, redesigning kickers, inventing new moves, and he’s ready for a new frontier.
“I just need to find a spacesuit!”
Original Source: NASA News Release