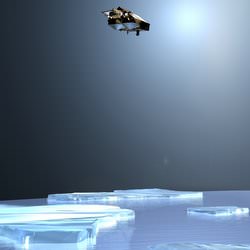
Artist’s illustration of Cryosat. Image credit: ESA. Click to enlarge.
The expectations of ice researchers across Europe are currently focused on a region of taiga woodland in Russia’s far north. Located in a forest clearing is Pad LC133 of Plesetsk Cosmodrome, where above the tree-line on a Rockot launcher stands ESA’s CryoSat satellite, due to start its flight into orbit this Saturday at 17:02 CEST.
The first of ESA’s Earth Explorer series – missions tailored to respond to particular needs of the Earth science community – CryoSat will use a specialised radar altimeter to measure changes in land and sea ice thickness over a three-year period, to provide a precise picture of how the Polar Regions are responding to climate change.
The generation of radar altimeters currently flying on satellites including ERS-2 and Envisat have made a large contribution to our knowledge of the mass balance of Greenland and Antarctic ice sheets, but they cannot return reliable data from the ice edge, where the rate of change is greatest. Similarly, over the ocean their resolution is insufficient to detect the majority of individual pack ice pieces. The design of CryoSat’s new SAR Interferometric Radar Altimeter (SIRAL) has been optimised to close these data gaps.
Many European ice specialists have played a part in the preparation for the mission, either through participation in the CryoSat Science Advisory Group, taking part in extensive in-situ calibration and validation activities in the Arctic and Antarctic, or preparing processing algorithms to turn raw altimetry results into usable information products. And whether or not they have made such direct contributions, researchers are eagerly awaiting the unique results CryoSat will return.
“Summer Arctic sea ice is shrinking ? but is it thinning?”
Dr Seymour Laxon of the Centre for Polar Modelling (CPOM) at University College London has been part of the mission with the start ? working closely with Lead Investigator Professor Duncan Wingham from the original mission proposal to ESA onwards.
“At that time we had just managed to extract the first plausible sea ice thickness maps from the radar altimeter on ESA’s ERS,” Dr. Laxon remembers. “Coupled with Duncan’s experience in mapping the ice sheets, ESA’s Earth Explorer Opportunity programme seemed like a great chance for us to build on what we had learned from the earlier ESA missions to design a mission that was really focused on altimetry over ice.”
A period of concentrated effort followed, as findings from past exploratory studies, and the latest results from ERS, were converted into a proposal for a new mission that could better those results.
“I vividly remember the selection procedure, with Duncan reporting back at each selection stage that CryoSat was still in the running,” Dr. Laxon adds. “I don’t think either of us were quite ready to hear the news that CryoSat was the first to be selected.
“At that point we both realised that the real work, to build a complete mission scenario from scratch, had started. Now everything is in place, the satellite sitting on top of its launcher, the processing system for the data, and plans for the post-launch validation campaign. Now we are just looking forward to seeing the very first data.”
In terms of his own area of interest, it is the sea ice data that Dr. Laxon is most looking forward to: “The most exciting thing for me is the prospect of seeing the first maps of sea ice thickness from CryoSat. We have not seen estimates of Arctic sea ice thickness around the North Pole since the last submarine data from 1999 were declassified.”
“Back then, analysis of this data suggested that a significant ? up to 40% – thinning had occurred since the 1960s, with the largest thinning around the pole. The big question is whether that thinning has reversed or continued as we have entered the new century.”
“That question has gained even more impetus since the news that the extent of summer ice in the Arctic has reached a record minimum this year. But has it also thinned? That’s the crucial question to which CryoSat will provide the answer.”
Opening a new window on the Poles
Professor Chris Rapley is Director of the Cambridge-based British Antarctic Survey and is also Chair of the Planning Group for the forthcoming International Polar Year, which will take place in 2007-8, during the time CryoSat will be carrying out its ice thickness survey. He has had a long involvement with radar altimetry over ice.
Prof. Rapley states: “I was deeply involved in the preparations for the ESA ERS and Envisat altimeters, and led or contributed to a substantial series of studies commissioned by ESA to explore the use of satellite radar altimeters over polar land ice and sea ice, and the technical advances required in instruments, data processing and analysis software to achieve useful scientific results.”
That activity included work on designing and implementing the UK-based ERS processing and archiving facility and the ESA altimeter data processing chain and associated software. Prof. Rapley also worked on design studies for more advanced altimeters along CryoSat’s lines.
A past member of ESA’s Earth Observation Advisory Committee (ESAC), Prof. Rapley was also involved in reviewing the CryoSat proposal and its adoption as an ESA Earth Explorer.
“What I am looking forward to is the best measure yet of the Antarctic and Greenland ice sheet mass balance,” Prof. Rapley adds. “CryoSat should also open a new window on the nature, geographic distribution and the seasonal/systematic behaviour of Antarctic and Arctic sea ice.”
“We can add ice thickness to our models”
Direct in-situ observations of land and sea ice have been necessary to establish that the CryoSat sensor will indeed ‘see’ as anticipated, and quantify residual geophysical uncertainties. As a member of ESA’s CryoSat Cal/Val Team, Dr. Christian Haas of the Alfred Wegener Institute in Bremerhaven coordinates German activities in this area.
“I lead the German CryoSat office,” says Dr. Haas. “It is the main interface between German users and scientists involved in Cryosat and ESA. We are also raising money in Germany for work with CryoSat.
“I am also coordinating the sea ice validation work in the Arctic and Antarctic. We at the Alfred Wegener Institute are the only group able to measure sea ice thickness directly, by helicopter-borne electromagnetic measurements with our EM-bird sensor. We have conducted the CryoVex (CryoSat Validation Exercise) campaign in 2003 and this year’s Bay of Bothnia campaign.”
As a geophysicist, Dr. Haas has been working with sophisticated software models of sea ice. Results from CryoSat will be used to first to check these models, then later be directly ingested within them to bring them closer to reality. The satellite’s ice thickness data in particular should literally add a new dimension to their representation of polar sea ice.
“As a scientist I am interested in using CryoSat data for validating our sea ice models, and combining the data with other met-ocean data to better understand the variability of sea ice thickness,” Haas explains. “We also want to assimilate sea ice thickness into our models.”
After CryoSat’s launch comes a further validation campaign, to compare the results from space to the reality on ground, as Haas adds: “I am looking forward to the validation of the satellite, and the opportunity of extending our airborne measurements laterally by means of the satellite.
Is Antarctic land ice growing or shrinking?
Other researchers, such as Dr. Massimo Frezzotti of Italy’s National Agency for New Technologies, Energy and the Environment (ENEA) in Rome hope to use the new satellite’s results to improve their knowledge of ice sheets on land.
Dr. Frezzotti has carried out in-situ studies of the Antarctic ice sheet between the Italian base of Terra Nova Bay on the shores of the Ross Sea and the new Franco-Italian Concordia base high on the Antarctic Plateau some 1200 kilometres inland. He also makes use of altimetry results in his research.
“I already use ERS altimeter data to study the influence of wind erosion on surface mass balance,” Dr. Frezzotti explains. “Previous altimeters are not able to provide a detailed model of the coastal areas, which are a very crucial area for mass balance studies. CryoSat will partially cover this gap.”
Satellite altimetry observations over the ocean have established a steady rise in global sea level of an average 0.3 millimetres a year. What is not known ? yet ? is how changes in polar ice thickness may be contributing to this trend.
“The Antarctic ice sheet contains sufficient ice to raise worldwide sea level by more than 60 metres if melted completely,” Dr. Frezzotti adds. “The amount of snow deposited annually on its surface is equivalent to five or six millimetres of global sea level. Thus the ice sheet could be a major source of water for the present-day rise in sea level, but the uncertainty is still large.
“Despite all available measurements of snow accumulation, ice velocity, surface and basal melting and iceberg discharge, it is still not known for certain even whether the ice sheet is growing or shrinking.” CryoSat should remedy this state of affairs.
Determining CryoSat’s orbit will improve its results
The Department of Earth Observation and Space Systems (DEOS) of the Delft University of Technology has an interest in the precise orbit determination (POD) of radar altimeter satellites. Because altimetry is based on the principle that time equals distance ? measuring how long it takes for a radar pulse to travel back from the Earth’s surface to the spacecraft – more exact knowledge of the satellite’s location at any one time greatly improves the quality and accuracy of the final data.
CryoSat has two onboard instruments for sharpening orbital estimates from a matter of metres down to a maximum three centimetres ? the Doppler Orbitography and Radiopositioning Integrated by Satellite (DORIS) radio receiver and a Laser Retro-reflector (LRR). Both these devices are found on a number of different satellites, and work based on global networks of radio transmitters and laser stations.
In addition a satellite trajectory prediction model will be created by DEOS to forecast how CryoSat’s orbit will be perturbed by the slight pressure of sunlight and the drag of the upper atmosphere as well as gravitational tugs from terrestrial gravity field anomalies as well as the influence of the tides, other planets and our Sun.
“We will determine CryoSat’s orbit, but in addition we will also perform cal/val activities for its SIRAL instrument, particularly in the Low-Rate Mode (LRM) over the open ocean and inland ice sheets,” said Dr. Ernst Schrama. “Comparing LRM sea surface results to in-situ buoys and tidal gauges should enable a means of externally validating LRM results.?
“We will also add CryoSat data to our Radar Altimeter Database System (RADS) compiled from other current as well as past altimeter missions. The database will be used to inter-compare the performance of SIRAL against other altimeters.”
Beyond improving the quality of CryoSat results, RADS also represents a scientific resource in its own right, which provides a continuous set of sea level measurements of constant quality. RADS can be used for scientific and operational oceanography as well as detecting slight variations in the Earth’s gravity field to infer its interior structure.
Original Source: ESA News Release