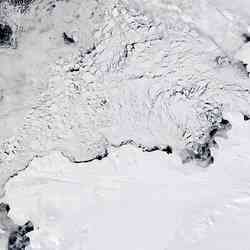
Antarctic ice sheets. Image credit: NASA Click to enlarge
Pamela Conrad, an astrobiologist with NASA’s Jet Propulsion Laboratory, has traveled to the ends of the Earth to study life. Conrad recently appeared in James Cameron’s 3-D documentary “Aliens of the Deep,” where she and several other scientists investigated strange creatures that inhabit the ocean floor.
On June 16, 2005, Conrad gave a lecture entitled, “A Bipolar Year: What We Can Learn About Looking for Life on Other Planets by Working in Cold Deserts.”
In part 1 of this edited transcript, Conrad describes what sort of signs we could look for to see if there is life in an alien environment.
“In the past three years, I’ve been engaged in a project with several of my colleagues that takes us to hot and cold deserts. We want to observe the signatures of life, and see if we can tell the difference between places where life is and where life isn’t. The reason we go to deserts is to cut down on the number of confounding variables that are introduced by all kinds of life. Basically, we don’t want to be scraping away the dog poop to find the bacteria in the dirt.
This past year we were privileged to go to both the Arctic and the Antarctic. So this is my bipolar year, and what we were doing there is relevant to space exploration because, like a desert, the conditions on the surface of other planets are very harsh.
We look at rocks because, if life had been and is already gone – in other words, it’s dead, or it’s so dead it’s been fossilized and altered – you can find that in the rock record.
To detect life anywhere, you need to be able to investigate the environment and find measurable clues. If it’s not something you can define in measurable terms, it’s not science. So by definition, we’re kind of out in the cold, so to speak.
One of the challenges is coming up with measurable terms by which you could define life. The terms have to be universal enough to not miss life on another planet, if it was unlike the life we have here. We have a sample set of one: the biosphere on the Earth. We try to use the knowledge we have about life here to come up with those terms, and so we try to think about life in the most general descriptive terms we can.
We look for life in places that are habitable; places that are capable of supporting life. But habitability is difficult to define, because we only have a vague notion of what makes an environment habitable. At NASA, we’re very big on looking for water as one of the facets of habitability.
Water is as important to life in the desert as it is to us. After a fresh snowfall, when rocks get heated up and melt the ice, you see a bloom of cyanobacteria on the surface of the rock. Yet they are able to maintain a minimal existence when there’s not much precipitation.
One reason metabolism has to slow down in the Antarctic winter is because the water is in a solid phase and it’s not accessible. Living things can only use ice when it melts and becomes a good solvent. Using ice is like using a mineral in the crystal phase — when it’s in the solid form, you’ve got to use some energy to bust up those bonds to do something with it. There are organisms in Antarctica that have antifreeze types of molecules in them, fish that possess molecules called glycoproteins. When an ice crystal forms in the fish, the molecule grabs hold of the ice crystal as it starts to grow, and doesn’t let it grow in the direction that its energetically most easily grown. Because it can’t grow, the ice crystal gives up the ghost and turns back into water.
Besides water, we think that certain kinds of chemical elements are important for life elsewhere. Life on Earth is made of carbon and hydrogen and phosphorus and a few other important things, and we need the oxygen in the air. But there are microbes on Earth that breathe metal, and they don’t care about oxygen.
So habitability is really habitable in the eyes of the beholder. When you’re defining it, you’ve got to think about the broadest set of terms you can in order to encompass any kind of life you might be able to imagine. The ultimate assessment of whether a place is habitable is, of course, to see if it is inhabited.
You ask one set of questions if you want to know, “Can I set up housekeeping here?” You might ask another set of questions if you want to know, “Is anybody home?” But at the heart of it all, whether or want to live there or just see if anyone’s home, you have to know something about the neighborhood. You’ve still got to do all the experiments that tell you about the geophysical, mineralogical, and atmospheric properties of the planet. If you’re looking for life, you’ve got to have some notion about what sort of thing you’re trying to support with that environment.
Erupting about 5 million years ago, from a series of fractures known as the Cerberus Fossae, the water flowed down in a catastrophic flood, collecting in an area 800 x 900 km and was initially an average of 45 meters deep. Click image for larger view.Credit: ESA/Mars Express
So what would constitute proof? If you want to say that something has been proven, you have to achieve a certain level of consensus in the scientific community, otherwise your peers will tear you into little bits and pieces in the literature. Of course, there is never a complete consensus: that’s why we nasty scientists fight with each other endlessly. But we have to at least come up with terms. We can agree or disagree with each other’s theories, but we have to agree on the terms and the measurements.
So what kind of measurements could we make if we were looking for life? Does a planet look different if life has been there? For example, if you go into my kitchen after I’ve eaten, you might see a plate or a crumb. That’s a clue that I was there. There are clues at the planetary level too. A biomarker – a clue that says life was there – can be anything that was produced by life. The clue can be chemical, because chemicals comprise everything. I am a sack of chemicals, just like this podium is a sack of chemicals. Just what chemicals there are, and in what proportion to each other, and how they’re arranged in 3-D, is what distinguishes me from this. It’s a simple way of distinguishing categories of things.
Chirality is a biomarker as well. What chirality means is that some molecules are mirror images of each other, and the living molecules tend to be a certain handedness. When it comes to amino acids, which are the constituents of the proteins that make up life, living things like to use the left-handed form. And when it comes to the sugars, living things like to use the right-handed form. There are exceptions to these, but that’s a general case.
Isotopes also can be a biomarker. Some molecules come in different isotopic flavors, where some are slightly heavier than others. Living things like the lighter variety, probably because it’s energetically less expensive to process.
Complex polymers also could be biomarkers. Of course, plastic is a complex polymer. The again, we made the plastic. So this whole distinction between natural and unnatural – if humans made it, it’s still biogenic. So think about that. My car is a biosignature. What kind, I’m not sure.
If you’re going to define life in measurable terms, I’d like to keep it really simple. You could define life by what it’s made of, or you could define life by what it does. I like to define life by what it’s made of, because as soon as you say the “does” word, you’re talking about a process. A process is something that happens through time. Then you’ve got to figure out what the sampling rate should be. How often should you look, and how long should the whole experiment take? A process is a little more problematic because it takes time, and you may be wrong about how often to look, or how long you should look for.
Processes – making stuff, reproducing, or evolving – can take place over different time scales. So if you’re only looking at processes, and you have two that are vastly different in their time scales, you won’t be able to do the same experiment to look at them both. So I like to look at life in terms of what it is. Not to say we couldn’t add in a little bit of process-based stuff, but when you look at what life is, it gets simple really fast. It’s unique chemistry, some kind of proportionate chemicals, arranged in some way, and the “arranged in some way” is what I call structure.
If I were looking for life on another planet or a moon, I would look for places where interesting chemistry could happen, so that the ultimate evolution of that chemistry could create a living system. I would think about places like Europa, which has an ocean beneath ice. I would think about other places where ice exists, like comets. I would think about Titan, Saturn’s moon. I would think about all those places where interesting chemistry occurs, because chemistry is clever. You can get all kinds of interesting molecules.
Original Source: NASA Astrobiology