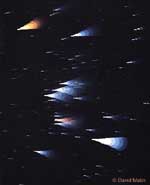
Image credit: David Malin
Monday, December 6 – If you’re up before dawn this morning, be sure to take a moment to step outside and have a look at the Moon and Jupiter. This morning the Moon will appear above and to the right of the “Mighty Jove”, but tomorrow morning they are going to be a whole lot closer! And speaking of closer, somewhere out there in the asteroid belt, it’s going to be a busy Monday because Asteroid 2002 YP2, Asteroid 12382 Niagara Falls and Asteroid 12397 Peterbrown all make their nearest approach to the Earth today.
For Northern Hemisphere observers, today Comet C/2004 Q1 (Tucker) is at perihelion, or the nearest point in its orbit of the Sun today. With an estimated magnitude of 10, Comet Tucker should be visible to both large binoculars and small telescopes sliding through Andromeda just a bit southwest of M31. As with all comets, activity increases as they near the Sun, so be on the lookout for coma or above average brightenings.
Tonight’s unusual treat is for the Southern Hemisphere viewer when the Phoenicid meteor shower reaches its peak. With an estimated fall rate of about 5 per hour, this particular shower might not seem very exciting, but it has an unusual place in history. In 1956 over 100 meteors per hour were recorded, marking the point of this shower’s discovery. The stream is believed to be the offspring of the long lost periodic comet Blanpain observed in 1819. Although the exact time of peak activity is currently unpredictable, past observations show this meteor shower begins right after sunset and radiates from constellation of Phoenix. It is unusual because it leaves very few visible trails but is well-known for its bright flashes and exploding fireballs! Lucky birds…
Tuesday, December 7 – Wake up North America! This morning is a grand occulation of Jupiter by the Moon. Encompassing Canada and the biggest majority of the United States (for you who are still warm? sorry… you’ll only get a grazing event.) the Moon will silently slide over Jupiter at the unholy hour of just before 4:00 a.m. Since timing is especially critical in these type of events, you must visit the IOTA predictions for precise times in your area. (relax Florida keys and south Texas, there’s times in there for your graze as well.) This provides a wonderful opportunity for photographs as well as just a spectacular event to witness. It is especially fitting since the Galileo spacecraft went into orbit around Jupiter on this day in 1995. So hug your loved one, hug your scope, hug your dog, or just watch Venus and Mars still hugging the horizon and enjoy!
While waiting on Jupiter to re-appear, those of you with large aperture telescopes might like to take the opportunity to view Comet Tsuchinshan at perihelion this morning. Although this comet has been holding a recent magnitude of 13, it may reach an estimated magnitude of 11 by this time. It has moved quietly across the southern border of Leo for the last few weeks and should be quite near Beta Leonis and the Coma Berenices border at this time.
On a side note, Apollo 17 was launched on this date in 1972, Gerard Kuiper belted his way into fame by being born on this day in 1905 and this is also the earliest sunset of the year. Astronomers? Let’s celebrate!
Wednesday, December 8 – If you are out this morning before dawn, take a moment to find bright Venus down on the horizon and work your way up the sky as Mars, the crescent Moon, Spica, and Jupiter form nearly a straight line across the winter skies.
Asteroid 4701 Milani, Asteroid 2004 RZ164, Asteroid 3353 Jarvis and Asteroid 2062 Aten all make their closest approach to the Earth today and Comet C/2004 S1(Van Ness) is at perihelion. At a rough magnitude of 16, this newly discovered comet will be in the range of professional observing. Ephemerides are provided by following the link (and I’ll see you at the observatory).
For binoculars and small telescopes, tonight would be a great opportunity to hunt down a southern Messier object in Capricornus. At magnitude 8, the M30 can be found just a bit over 6 degrees south of Gamma Capricorni. To smaller aperture telescopes and large binoculars, this 40,000 light year distant globular cluster will appear small and faint, but larger telescopes will see a dense, bright core and splendid resolution. As an added bonus, 41 Capricorni (in the same field of view) is a double star!
Thursday, December 9 – Looking for an unusual photo opportunity? Then check out Mars this morning as it appears almost between the very slender crescent Moon and Venus. Coupled with “earth shine” this trio will be very low on the horizon!
While we’re enjoying early evening dark skies, the window opens for binoculars and small telescopes to locate one of the largest and most spectacular of the southern globular clusters – the M2! By heading north/northeast of Beta Aquarii (remember this star – we’ll use it again), the 6th magnitude M2 (NGC7089) will show to small aperture scopes as well as binoculars and turn into stunning resolution in the large telescope. Cataloged by Messier 1760, this 150 light year wide “ball of stars” might seem less impressive than the M13, but at 50,000 light years in distance it’s significantly further away!
Southern Hemisphere viewers, you’re in luck again! Tonight will be the maximum of the Puppid-Velid meteor shower. With an average fall rate of about 10 per hour, this particular meteor showing could also be visible to those far south enough to see the constellation of Puppis. Very little is known about this meteor shower except for the streams and radiants are very tightly bound together. Since studies of the Puppid-Velids are just beginning, why not take the opportunity to watch? Viewing will be all night long and although most of the meteors are faint, it is known to produce an occasional fireball.
Friday, December 10 – Do you think you can catch Luna one last time? Then try again this morning as the Moon will make its final dawn appearance very close to the horizon. Just above it will be Venus, and higher to the left will be tiny Mars. Missing Mercury is now at perihelion – its closest approach to the Sun – but it is also at inferior conjunction, making it invisible to us because it is hiding between the Earth our “nearest star”.
Tonight will be the peak of the Monocerotid meteor shower. Here again we have an example of an obscure and unstudied shower because no one is sure of exactly where the precise radiant is located. By keeping watch loosely on the constellations of Gemini and Monoceros, you may see a few of these faint and fast meteors at a rate of 3-12 per hour. Who knows? Perhaps one of these strange meteors may have been responsible for the observed fall in 1984 that hit a Claxton mailbox!
While out viewing tonight, take advantage of the moonless early evening to try hunting down a very large and elusive galaxy that can be spotted with binoculars and even unaided from a very dark observing site. The M33, or “Triangulum Galaxy” is very misty, vague and also a totally wonderful galaxy for study. Just west of Alpha Triangulum, this galaxy is about the size of the full Moon, but it is so diffuse it’s sometimes hard to locate. Cataloged by Charles Messier in August 1764, the M33 is often known as the “Pinwheel”, because of its distinguishable arm structure. As a part of our local galaxy group, the M33 (NGC598) is quite prized by amateurs for its ability to resolve. It has a distinct concentration toward the nucleus area plus a northern and southern “arm” that are within a small telescope’s capabilities. Telescopes ranging between 12.5″ to 16″ and larger will find a wealth of NGC and IC objects hiding within this fantastic galaxy, allowing us to study star clusters and nebula almost 750,000 light years away. It’s out there tonight!
Saturday, December 11 – For those of you who like to get up early to capture space oddities, this morning Mars will occult TYC 6174-00681-1 (10.2 magnitude star).
On this date in 1863, Annie Jump Cannon was born. She was a United States astronomer who created the modern system for classifying stars by their spectra. Why not celebrate this achievement by coming along with me and viewing some very specific stars that have unusual visual spectral qualities! Let’s grab a star chart, brush up on our Greek letters and start first with Mu Cephei. Nicknamed the “Garnet Star”, this is perhaps one of the most red stars visible to the unaided eye. At around 1200 light years away, this spectral type M2 star will show a delightful blue/purple “flash”. If you still don’t perceive color, try comparing Mu to its bright neighbor Alpha, a spectral type A7, or “white” star. Perhaps you’d like something a bit more off the beaten path? Then head for S Cephei about halfway between Kappa and Gamma toward the pole. Its intense shade of red makes this magnitude 10 star an incredibly worthwhile hunt.
To see an example of a B spectrum star, look no further than the Plieades. All the components are blue white. Want to taste an “orange”? Then look again at Aldeberan, or Alpha Tauri, and say hello to a K spectrum star. Now that I have your curiosity aroused, would you like to see what our own Sun would look like? Then chose Alpha Aurigae, better known as Capella, and discover a spectral class G star that’s only 160 times brighter than the one that holds our solar system together! If you’re enjoying the game, then have a look at one of the most unusual spectral stars of all – Theta Aurigae. Theta is actually a B class, or a blue/white but instead of having strong lines in the helium, it has an abnormal concentration of silicon, making this incredibly unusual double star seem to glitter like a “black diamond”.
Still no luck in seeing color? Don’t worry. It does take a bit of practice! The cones in our eyes are the color receptors and when we go out in the dark, the color-blind rods take over. By intensifying the starlight with either a telescope or binoculars, we can usually excite the cones in our dark-adapted eyes to pick up on color. While you’re out tonight waiting on the meteor shower, try watching Orion. it contains several stars of different spectra! For the Southern Hemisphere, have a look at the Southern Cross as well. Awesome!
Tonight will also be the peak of the Sigma Hydrid meteor shower. With its radiant near the bright star, Procyon, this will make an nice “all hemispheres” type of show. But don’t hold your breath… The Sigma Hydrids are famous for being both notoriously fast and faint. With a predicted fall rate of about 3-12 per hour at maximum, this is not a spectacular show, but thanks to the new Moon tonight, you might stand a chance at catching a meteor that is normally only observed by the most serious of sky watchers.
Sunday, December 12 – Today the Moon at perigee and will be about 358,002 km or 222,452 miles away from Earth. If this make you feel like singing the “blues”, then why don’t we take advantage of dark skies and hunt down two very unique planetary nebulae tonight!
Our first will be the NGC7662, more commonly known as “The Blue Snowball”. Starting in the constellation of Andromeda, you will find this unique nebula by locating Iota and Omicron Andromedae and identifying the fifth magnitude star 13, about one-third the distance between them. The NGC7662 is approximately one-half a degree southwest. Shining at a decent magnitude 8, the “Blue Snowball” is achievable in large binoculars and very small telescopes, but due to its size, it will appear almost stellar. By upping the magnification you will find the NGC7662 to be a wonderfully blue “disc” that has a central star that can be spotted with larger telescopes. At around 5600 light years away, this unusual character is very similar to our next study!
Moving on to the constellation of Aquarius, recall our earlier study of the M2 and re-locate Beta. Now hop southwest to bright star, Nu. Only one degree west of Nu you will find the NGC7009, or the “Saturn Nebula”. Also shining at a magnitude 8, the NGC7009 can be spotted in large binoculars and small scopes, but requires the use of high power to understand “why” it is so named. Larger instruments will easily reveal that the NGC7009 has two extensions, or “lobes” that make it appear visually like the planet Saturn! As a high surface brightness object, its central star is also a extremely hot blue dwarf that emits a continuous spectrum. Large telescopes can reveal a substantial amount of detail in this particular planetary nebula, but most simply enjoy it for its unique shape and “electric” coloration. Best of luck!
(Be aware that the most prolific of all meteor showers – the Geminids – peaks on December 13. Observers may wish to start their vigil before dawn tomorrow! Full information will be provided in next week’s issue.)
Until next week fellow SkyWatchers, keep looking up and thinking – the Universe is out there waiting to be explored! Wishing you clear skies and light speed… ~Tammy Plotner