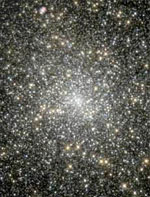
Image credit: Hubble
Monday, November 8 – Saturn turns retrograde on this date and today will be the closest approach of asteroid 4433 Goldstone to Earth at a respectable distance of 1.358 AU – or just 149,597,871 km. While asteroids of this nature are being closely monitored, they aren’t really observable to the majority of the amateur astro community. In case you’ve ever wondered just what it would be like to follow an asteroid, why not try your hand at locating and tracking one one of the brightest and easiest for beginners? Vesta!
Asteroid Vesta is considered to be a minor planet since its approximate diameter is 525 km (326 miles) wide, making it slightly smaller in size than the state of Arizona. Vesta was discovered on March 29, 1807 by Heinrich Olbers and it was the fourth such “minor planet” to be identified. Olbers discovery was fairly easy because Vesta is the only asteroid bright enough at times to be seen unaided from Earth. Why? Orbiting the Sun every 3.6 years and rotating on its axis in 5.24 hours, Vesta has an albedo (or surface reflectivity) of 42%. Although it is about 220 million miles away, pumpkin-shaped Vesta is the brightest asteroid in our solar system because it has a unique geological surface. Spectroscopic studies show it to be basaltic, which means lava once flowed on the surface. (Very interesting since most asteroids were once though to be rocky fragments left-over from our forming solar system!)
Studies by the Hubble telescope confirmed this, as well as a large meteoric impact crater which exposed Vesta’s olivine mantle. Debris from Vesta’s collision then set sail away from the parent asteroid. Some of them remained within the asteroid belt near Vesta to become asteroids themselves with the same spectral pyroxene signature, but some escaped through the “Kirkwood Gap” created by Jupiter’s gravitational pull and allowed these small fragments to be put into an orbit that would eventually bring them “down to Earth”. Did one make it? Of course! In 1960 a piece of Vesta fell to Earth and was recovered in Austrailia. Thanks to Vesta’s unique properties, the meteorite was definitely classified as once being a part of our third largest asteroid.
Now, that we’ve learned about Vesta, let’s talk about what we can see from our own backyards. As you can discern from the image, even the Hubble Space Telescope doesn’t give incredible views of this bright asteroid. What we will be able to see in our telescopes and binoculars will closely resemble a roughly magnitude 7 “star”, and it is for that reason that I strongly encourage you to visit Heaven’s Above, follow the instructions and print yourself a detailed map of the area. When you locate the proper stars and the asteroid’s probable location, mark physically on the map Vesta’s position. Keeping the same map, return to the area a night or two later and see how Vesta has moved since your original mark. Since Vesta will stay located in the constellation of Aquarius all month, your observations need not be on a particular night, but once you learn how to observe an asteroid and watch it move – you’ll be back for more!
Tuesday, November 9 – Remember last week when Jupiter and Venus did a spectacular morning dance in the sky? Well, the excitement hasn’t ended yet for now the Moon has joined the show. Before local dawn, Jupiter will be 1 degree to the lower right of the crescent Moon. For most of us, this beautiful “sky scenery” would be pleasure enough, but for those living in eastern Canada and the north-eastern United States, something just a bit more exciting is about to happen – the Moon is going to occult Jupiter during the daylight hours! Timing for such events is very critical and varies widely by location. To ensure success, please visit the International Occultation Timing Association (IOTA) for precise times in your area.
Asteroid 2000 JE5 will be performing a very near Earth fly-by today as it passes only 0.131 AU away. While that is less than the distance between Earth and the Sun, it is still 19,597,338 km or 12,177,221 miles away!
Thanks to dark skies, early tonight would be a great opportunity to use telescopes or large binoculars to study one of the finest of deep space objects, the “Ring” nebula. Located in the quickly westering constellation of Lyra and roughly halfway between bright stars Sheilak (Beta Lyrae) and Sulfat (Gamma Lyrae) this wonderful planetary nebula can be seen in small binoculars and comes to life with a telescope. But before we view the “Ring”, let’s learn a little more about the M57 and the two stars we’ll use to find it.
Beta is a variable star that averages around 3.38 magnitude at its maximum, but drops to around 4.1 at minima. This typical lyrid-type eclipsing variable is relatively easy to observe even without optical aid because nearby Gamma remains a constant magnitude 3.25. For a few days, both stars will appear to be about the same brightness, but about every 13 days, Sheilak will fade out to about half the brightness of Sulafat! For those of you aiming a telescope towards Gamma, you will find that it is a optical double star with a 10th magnitude companion.
Roughly halfway between these two interesting stars (but a bit closer to Beta) is tonight’s object. The M57 is a classic example of a planetary nebula first discovered by French astronomer Antoine Darquier in 1779 and cataloged only days later by Charles Messier. At approximately 2300 light years away, the “Ring” is basically the ejecta of a dying star. Many theories exist about the structure of the nebula itself , but popular opinion is that we may be looking through the shell, much like looking down the barrel of a gun. Its interior star has reached white dwarf stages, slowly shedding its mass and complex waves of ultra-violet radiation which fluoresce the rarefied gases of the nebula expanding at the gentle rate of around 19 km (12 miles) per second. The nebula itself exhibits many different spectral qualities as seen in photography, but what does it really look like?
To binoculars, the M57 will appear almost stellar in size, but the small disk lacks the properties of starlight. To the average telescope, the “Ring” will appear much as you see here – a softly glowing torus with a gentle grey/green color. At low power it is spectacular because the accompanying stellar field is so rich. Larger telescopes can resolve the central star under excellent sky conditions along with variances in the structure of the ring itself. Reach for the “Ring” tonight… You’ll be glad you did!
(In loving memory of Carl Sagan who was born on November 9, 1934. You were an inspiration to us all…)
Wednesday, November 10 – The early morning show continues as Jupiter, Venus, the very thin crescent Moon and Mars all appear with 20 degree of each other just before local dawn. For observers in other parts of the world, today is your day to observe an occultation as the Moon moves across Venus! The “footprint” for this occultation will be for skywatchers in Australia, New Zealand and Southern Asia. As always, timing is everything, so please visit IOTA for precise times for your locations. Observers with sense of curiousity and a large telescope might like to know that Mars will also occult an 11.8 magnitude star. (The challenge will be seeing how long you can follow the progress.) Information on this event is slim, but if you want to know where and when – here’s your clue.
This evening we are once again going to study a single star and it will help you become acquainted with the constellation of Perseus. Its formal name is Beta Persii and it is the most famous of all eclipsing variable stars. Tonight, let’s identify Algol and learn all about the “Demon Star”.
Ancient history has given this star many names. Associated with the mythological figure, Perseus, Beta was considered to be the head of Medusa the Gorgon, and was known to the Hebrews as Rosh ha Satan or “Satan’s Head”. 17th century maps labeled Beta as Caput Larvae, or the “Spectre’s Head”, but it is from the Arabic culture that the star was formally named. They knew it as Al Ra’s al Ghul, or the “Demon’s Head”, and we know it as Algol. Because these medieval astronomers and astrologers associated Algol with danger and misfortune, we are led to believe that Beta’s strange visual variable properties were noted throughout history.
Italian astronomer Geminiano Montanari was the first to note that Algol occasionally “faded” and its methodical timing was cataloged by John Goodricke in 1782, who surmised that it was being partially eclipsed by a dark companion orbiting it. Thus was born the theory of the “eclipsing binary” and it was proved spectroscopically in 1889 by H.C. Vogel. At 93 light years away, Algol is the nearest eclipsing binary of its kind and is treasured by the amateur astronomer for it requires no special equipment to easily follow its stages. Normally Beta Persii holds a magnitude of 2.1, but approximately every three days it dims to magnitude 3.4 and gradually brightens again. The entire eclipse only lasts about 10 hours!
Although Algol is known to have two additional spectroscopic companions, the true beauty of watching this variable star is not telescopic – but visual. The constellation of Perseus is well placed this month for most observers and appears like a glittering chain of stars that lay between Cassiopeia and Andromeda. To help further assist you, re-locate last week’s study star, Gamma Andromedae (Almach) east of Algol. Almach’s visual brightness is about the same as Algol’s at maxima. Tonight at 16:55 UT, Algol will be at minima and will appear approximately the same brightness of Alpha Trianguli. Depending on what time zone you live in, it would be possible for you to see Algol return to full brightness once again at 02:55 UT on November 11! If you are clouded out, don’t worry. Agol reaches minima again on November 13 at 13:44 UT, November 16 at 10:33 UT, November 19 at 7:22 UT, November 22 at 4:11 UT, November 25 at 1:00 UT, November 27 at 21:49 UT and November 30 at 18:38 UT. Just remember that it only takes 10 hours to complete its eclipse and enjoy the “Demon”!
Thursday, November 11 – Southern hemisphere viewers? You asked for it and you got it. This morning the Moon will occult Mars for East Africa and Australia! It was a bit difficult for me to find precise timing information for you, but I did locate at list of cities and times that you might find useful. Best of luck!
Uranus becomes stationary today and Comet P/1996 R2 (Lagerkvist) will make its closest approach to Earth today at a distance of 1.793 AU. At around magnitude 17, this would be one serious observing challenge!
Tonight let us take the opportunity to visit with another planetary nebula seen from a different perspective – “The Dumbbell”. You will want to start fairly early, because as with Lyra, the constellation of Vulpecula is fast declining. The M27 is challenging with small binoculars, readily apparent in larger ones and superior in even small telescopes. By using previously visited stars Altair and Albireo, look for four stars that form the constellation of Sagitta between them. On a good night, the “Arrow” is easy to recognize. By looking at this constellation, get in mind the distance between the arrow’s point, Gamma, and the first of the three stars that make the arrow’s tailfeathers. Using this as your measure, return to Gamma and move the same distance due north, and let’s learn about the M27!
The M27 was the first planetary nebula discovered by Charles Messier and cataloged on July 12, 1764. As we learned with the “Ring”, a planetary nebula is a star shedding its mass in a thin, cold field of hydrogen and helium gas illuminated by the energy of the star itself. It is the strong ultraviolet radiation that excites these rarefied gases to glow in the soft greenish-blue that our eyes can perceive in a spectral condition which can only exist in space – doubly ionized oxygen! The nebula lies about 1,000 light years away from us and is expanding at a rate of about 17 miles per second, meaning that it grows about one arc-second per century. If these figures are correct, it has taken about 50,000 years for the M57 to have reached it’s present size.
The Hubble Telescope reveals the M27 in all its glory. Instead of looking through the planetary’s shell as we did with the M57, we are looking at the entire structure itself. Larger telescopes will have no problem resolving out tenuous rifts, folds and concentrations in the lobes of the nebula, as well as embedded stars. The central star is also evident in larger telescopes and the outer shell named the Millikin 1976 is apparent in Earth based telescopes with an aperture of around 30″. But what about large binoculars and the average backyard telescope?
Don’t worry. The wonderful “dumbbell” shape first described by John Herschel is very there. The spectral qualities described above are easily seen in the most modest of instruments! The M27 is perhaps one of the finest of deep sky objects for the amateur, and tonight? It’s yours…
Friday, November 12 – This morning will mark the peak of the Southern Taurid meteor shower. The Earth will be entering the second “stream” of debris in the early morning hours. The Taurids have a predicted fall rate of 7 per hour, but thanks to their relatively slow speed (27 km or 17 miles per second) and a New Moon, they might produce spectacular results. Good luck!
Asteroid 33342 (1998 WT24) will make a near Earth fly-by as it passes on 0.097 AU away. Hey, wait a minute. That dry fact seems pretty close doesn’t it? Then let’s find out… 0.097 AU would be 14,511,006 km or 9,016,721 miles. That’s roughly 34 times further away than our Moon, yet less than half the distance to our nearest planet, Venus. In astronomical terms? That is close!
Tonight we continue with our planetary studies by finding another such nebula located within a deep-space object. The M15 is well positioned now in the constellation of Pegasus and we start by once again identifying the “Great Square”. Leading the constellation to the west of the square is bright star Epsilon Pegasi, or Enif. By focusing either small binoculars or your telescope on Epsilon, you will know if you have the correct star, for Enif appears gently red. From there, the M15 is an easy catch in binoculars about 4 degrees northwest (about one field of view) and will appear to modest powers (5X30) as a small, round fuzzy patch with a star caught on the edge. Now let’s use a telescope and learn about the M15 as we view it.
Discovered originally by Miraldi in 1746, the wonderfully compact globular cluster was rediscovered by Charles Messier in 1764. It is one of the richest of clusters with an intense, compact core region and ranks as the 12th brightest globular in the sky. Its thousands of stars are gathered in a huge ball spanning 120 light years across and approximately 40,000 light years from Earth, but the M15 has many surprises. It has well been known this particular globular cluster contains many variable stars and pulsars, as well as a planetary nebula. As a rich radio x-ray source, studies of the M15 revealed many neutron stars and made headlines when Chandra revealed the presence of a binary neutron star.
To the average telescope, is simply a beautiful compact globular cluster. Even small apertures will begin to resolve out individual stars. For those with larger telescopes, take the time to “power up” on the M15 and find the planetary amidst the awesome resolvability!
Saturday, November 13 – Double your pleasure, double your fun, as tonight we’ll view two star clusters instead of just one! It’s a Saturday night and what finer way to celebrate than to view one of the most impressive star clusters in our galaxy – the NGC869 and NGC884. This pair of rich galactic open clusters are a naked-eye object from a dark site, easily seen in the smallest of binoculars from urban locations and beyond compare when viewed with a telescope at lowest power.
The western-most of the pair is NGC869, also known as “h Perseii”. It contains at least 750 stars clustered in a brilliant mass spanning about 70 light years, and approximately 7,500 light years away from us. It’s eastern companion is NGC884, or “Chi Perseii”. The statistics are almost a match, but NGC884 only has about half as many stars – some being “super giants” over 50,000 times brighter than our own Sun! These twin clusters have only one major difference: NGC884 is approximately 10 million years old and the NGC869 is perhaps 5 million. The existance of these splendid clusters was cataloged as far back as 350 B.C. with both Ptolemy and Hipparchus noting their appearance – yet Messier never “discovered” them!
Be sure to check out Algol again tonight, it’s minima is at 13:34 UT. Mercury will also be occulted by the Moon today, but it is far to close to the Sun to observe.
Sunday, November 14 – Tonight the Moon is at perigee, or the closest in its elliptical orbit to Earth. The challenge this evening will be to spot the very slender two-day old crescent while it is at its closest – only 356,410 km (221,473 miles) away!
This would be extremely fitting as we observe the 35th anniversary of the Apollo 12 mission. At 11:20:00 a.m. EDT, from launch complex 34-A at Kennedy Space Center, Florida, the Apollo 12 left Earth on November 14, 1969 in the second manned space mission bound for the Moon.
For Southern Hemisphere observers, tonight would be a great opportunity to study the Small Magellanic Cloud. At 210,000 light years away, this near neighbor to the Milky Way will be apparent to the naked eye just north of Beta Toucanae. Easily viewed in binoculars and incredible in telescopes, the Small Magellanic Cloud is home to the rich globular cluster 47 Toucanae. As the second brightest globular cluster in the sky, 47 was once believed to be a star until the 1750’s when French astronomer Nicohlas Louis du Lacaille discovered its true nature.
Until next week? Keep looking up… I wish you clear skies and light speed!
~Tammy Plotner