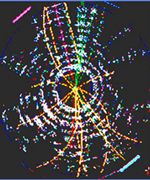
Image credit: Berkeley Lab
In a case of the plot thickening as the mystery unfolds, the Higgs boson has just gotten heavier, even though the subatomic particle has yet to be found. In a letter to the scientific journal Nature, published in the June 10, 2004 issue, an international collaboration of scientists working at the Tevatron accelerator of the Fermi National Accelerator Laboratory (Fermilab), report the most precise measurements yet for the mass of the top quark ? a subatomic particle that has been found ? and this requires an upward revision for the long-postulated but still undetected Higgs boson.
“Since the top quark mass we are reporting is a bit higher than previously measured, it means the most likely value of the Higgs mass is also higher,” says Ron Madaras, a physicist with the U.S. Department of Energy’s Lawrence Berkeley National Laboratory (Berkeley Lab), who heads the local participation in the D-Zero experiment at the Tevatron. “The most likely Higgs mass has now been increased from 96 to 117 GeV/c2” ? GeV/c2 is a common particle-physics unit of mass; the mass of the proton measures about 1 GeV/c2 ? “which means it’s probably beyond the sensitivity of current experiments, but very likely to be found in future experiments at the Large Hadron Collider being built at CERN.”
The Higgs boson has been called the missing link in the Standard Model of Particles and Fields, the theory that’s been used to explain fundamental physics since the 1970s. Prior to 1995 the top quark was also missing, but then the experimental teams working at the Tevatron’s two large detector systems, D-Zero and CDF, were able to discover it independently.
Scientists believe that the Higgs boson, named for Scottish physicist Peter Higgs, who first theorized its existence in 1964, is responsible for particle mass, the amount of matter in a particle. According to the theory, a particle acquires mass through its interaction with the Higgs field, which is believed to pervade all of space and has been compared to molasses that sticks to any particle rolling through it. The Higgs field would be carried by Higgs bosons, just as the electromagnetic field is carried by photons.
“In the Standard Model, the Higgs boson mass is correlated with top quark mass,” says Madaras, “so an improved measurement of the top quark mass gives more information about the possible value of the Higgs boson mass.”
According to the Standard Model, at the beginning of the universe there were six different types of quarks. Top quarks exist only for an instant before decaying into a bottom quark and a W boson, which means those created at the birth of the universe are long gone. However, at Fermilab’s Tevatron, the most powerful collider in the world, collisions between billions of protons and antiprotons yield an occasional top quark. Despite their brief appearances, these top quarks can be detected and characterized by the D-Zero and CDF experiments.
In announcing the D-Zero results, experiment cospokesperson John Womersley said, “An analysis technique that allows us to extract more information from each top quark event that occurred in our detector has yielded a greatly improved precision of plus or minus 5.3 GeV/c2 in the top mass measurement, compared with previous measurements. The new measurement is comparable to the precision of all previous top quark mass measurements put together. When this new result is combined with all other measurements from both the D-Zero and CDF experiments, the new world average for the top mass becomes 178.0 plus or minus 4.3 GeV/c2.”
The D-Zero detector system consists of a central tracking detector array, a hermetic calorimeter for measuring energy, and a large solid-angle muon detector system. Berkeley Lab designed and built the two electromagnetic end-cap calorimeters and also the initial vertex detector, the innermost component of the tracking system. Tracking detectors supplement calorimeters by measuring particle trajectories. Only when trajectory and energy measurements are combined can scientists identify and characterize particles.
While raising the central value for the top quark mass appears to diminish the possibility that the Higgs boson could be discovered at the Tevatron, it does open a wider door for new discoveries in supersymmetry, also known as SUSY, an extension of the Standard Model that unites particles of force and matter through the existence of superpartners (sometimes referred to as “sparticles”). Supersymmetry seeks to fill gaps left by the Standard Model.
“The current mass limits or bounds that exclude supersymmetric particles are very sensitive to the top quark mass,” says Madaras. “Since the top quark mass is now higher, these limits or bounds are not as severe, which increases the chance of seeing supersymmetric particles at the Tevatron.”
Scientists from nearly 40 US universities and 40 foreign institutions contributed to the data analysis reported in the letter to Nature by the D-Zero experimental group. Berkeley Lab co-authors of the letter in addition to Madaras were Mark Strovink, Al Clark, Tom Trippe, and Daniel Whiteson.
Fermilab Director Michael Witherell said in a statement that these results do not end the story of precision measurements of the top quark mass. “The two collider detectors, D-Zero and CDF, are recording large amounts of data in Run II of the Tevatron. The CDF collaboration has recently reported preliminary new measurements of the top mass based on Run II data. The precision of the world average will improve further when their results are final. Over the next few years, both experiments will make increasingly precise measurements of the top quark mass.”
Fermilab, like Berkeley Lab, is funded by the Department of Energy?s Office of Science. In response to the Nature letter from the D-Zero group, Raymond L. Orbach, Director of the Office of Science, said: ?These important results demonstrate how our scientists are applying new techniques to existing data, producing new estimates for the mass of the Higgs boson. We eagerly await the next round of results from the vast quantities of data that are generated today at the Fermilab Tevatron.?
Berkeley Lab is a U.S. Department of Energy national laboratory located in Berkeley, California. It conducts unclassified scientific research and is managed by the University of California. Fermilab is a national laboratory funded by the Office of Science of the U.S. Department of Energy, operated by Universities Research Association, Inc.
Original Source: Berkeley Lab News Release